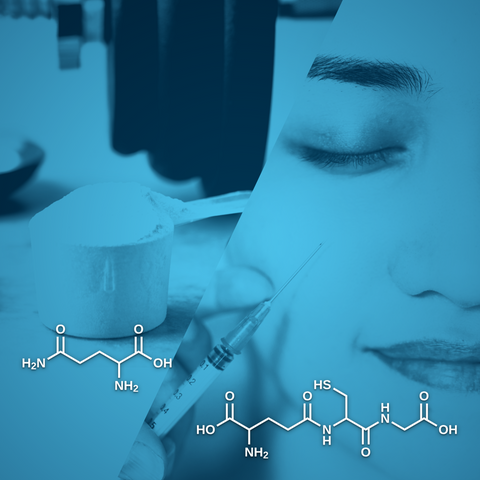
Glutathione is an antioxidant tripeptide found in nearly all eukaryotes that results from cysteine, glycine, and glutamic acid [1]. It is present at the same concentration as glucose and cholesterol in most cells. Such a high level highlights its importance, especially when considering the metabolic activity required for its production. In addition, its cellular and mitochondrial levels directly correlate with health and longevity. Glutathione exists in two forms: reduced (GSH) and oxidized (GSSG), the latter formed when two GSH molecules link through their sulfur atoms [2]. Reactive oxygen species, such as hydrogen peroxide (H₂O₂), can be neutralized by GSH peroxidases, which catalyze the conversion of H₂O₂ into water (H₂O) while oxidizing GSH to GSSG. The glutathione reductase then regenerates GSH from GSSG, maintaining the redox balance in the cell [3]. The ratio between the reduced and oxidized forms determines the cell's redox status. Healthy cells have a GSH/GSSG ratio above 100 at rest, while cells exposed to oxidative stress have a ratio between 1 and 10. The GSH-GSSG pair acts as the redox buffer within the glutathione system, preserving optimal redox balance in subcellular structures to the entire organism [4].
We've previously discussed glutamine and glutamate, as well as glutamate and GABA, in previous articles. In this article, we'll focus on the relationship between glutamine and glutathione, specifically their interplay and glutathione's roles and therapeutic applications.
Glutathione synthesis
In the cell, glutathione de novo synthesis occurs in the cytosol before being pumped into mitochondria [2]. The synthesis involves two steps. Cysteine and glutamate are first ligated by the glutamate-cysteine ligase. Next, the glutathione synthetase adds one glycine. While cysteine, glycine, and glutamine are all amino acids, glutamate is derived from glutamine. Glutamine is first transported into the cell and then into the inner mitochondrial membrane via specific transporters before being converted into glutamate by mitochondrial glutaminase. When glutamate goes to the cytosol, it can participate in glutathione biosynthesis [5]. At the organ level, the liver produces most of the circulating glutathione [6-8].
Importance of glutamine
Glutamine is a rate-limiting factor in glutathione synthesis [9,10]. Experiments in animals with uniformly labeled 13C-glutamine demonstrated that glutathione was enriched with five 13C atoms, indicating that glutamine is the primary source for glutathione synthesis [11]. Indeed, glutamine is the direct source of glutamate necessary for the first step of glutathione synthesis. It is also indirectly responsible for cystine uptake via the cystine/glutamate antiporter system that reduces to cysteine, another rate-limiting precursor in glutathione synthesis [12]. In addition, glutamine starvation triggers the impairment of the cystine/glutamate antiporter system and decreases glutathione levels in the cell [13].
Glutathione degradation
The γ-glutamyl transferase catalyzes GSH degradation [14]. At the body level, kidneys, lungs, and intestines consume most of the circulating GSH [15], highlighting the important oxidative stress that might occur in these organs [1].
Glutathione role
Glutathione has many functions through its redox-active sulfhydryl group (-SH). It is crucial for reducing oxidative stress, sustaining redox balance, supporting metabolic detoxification, and modulating the immune system [16].
Antioxidant defense
The glutathione redox system is the most critical antioxidant defense system in cells. It can directly scavenge diverse oxidants such as superoxide anion, nitric oxide, hydroxyl radical, and carbon radicals through the oxidation of GSH to its oxidized form, GSSG. It also detoxifies hydroperoxides, peroxynitrites, and lipid peroxides [17]. Glutathione also protects cells through vitamin C recycling [2]. In addition, GSH depletion and GSSG accumulation due to oxidative stress induce apoptosis by activating the SAPK/MAPK pathway [18]. Adequate glutamine levels support glutathione production, enhancing the body’s ability to counteract oxidative damage. It is especially critical in tissues with high oxidative stress.
Xenobiotic and endogenous compounds detoxification
Glutathione is involved in detoxification via its redox-active sulfhydryl group (-SH). It directly neutralizes persistent organic pollutants and promotes their excretion from the body [1]. It also protects from mercury, other toxic metals, and alcohol [19,20]. Glutathione participates in the plasma transport of toxins and xenobiotics mainly via the formation of glutathione S-conjugates, then excreted in the bile. This GSH conjugation is spontaneous or enzymatic via GSH-S-transferases [21,22].
Immune function
Glutathione is also involved in innate cell-mediated defense [23]. Its concentration directly regulates the balance between Th1 and Th2 T-helper cell subtypes and impacts host immunocompetence. High levels of GSH increase interleukin-12, a cytokine involved in T-cell progenitor’s differentiation into the Th1 subtype. Th1 cell dominance increases interferon-γ and interleukin-2, promoting Th1 cell proliferation and enhancing macrophage-mediated phagocytosis [24].
Glutathione in diseases
Altered glutathione levels may contribute to the onset and progression of various diseases, with GSH depletion being a common feature across many conditions [25]. Disrupted glutathione function in the brain has been associated with neuronal loss, both as part of the natural aging process and in neurological diseases like Alzheimer’s [26] and Parkinson’s diseases [27]. GSH imbalance and depletion are reported in many other brain disorders, including autism [28,29], bipolar disorder [30], schizophrenia [31], amyotrophic lateral sclerosis [32], and multiple sclerosis [33]. GSH depletion also occurs in pulmonary diseases (chronic obstructive pulmonary disease, asthma, and acute respiratory distress syndrome) and immune diseases such as HIV and autoimmune diseases. GSH depletion is also strongly associated with aging. In older people, higher glutathione levels correlate with better health and fewer illnesses [34].
Glutathione therapeutic applications
Due to glutathione depletion in many diseases, clinical trials have explored ways to enhance its synthesis, including administering its precursors (mainly N-acetyl-cysteine but also L-glutamine) or providing glutathione intravenously. Clinical trials reported positive results in a wide range of applications, including Parkinson’s disease [35], bronchiectasis [36], metabolic dysfunction-associated steatotic liver disease [37], exercise-induced fatigue [38,39], lead exposure [40], and healthy aging [41,42].
Conclusion
Glutathione is vital for overall health and longevity, playing roles in antioxidant defense, detoxification, and immune function. Maintaining adequate glutathione levels depends on the availability of glutamine and cysteine, two crucial amino acids needed for its synthesis. Together, they support the body's ability to neutralize oxidative stress, eliminate toxins, and maintain a strong immune response.
References
[1] Li, F., Li, S., Shi, Y., Lin, F., Rui, L., Shi, J. et al. (2024) Glutathione: A Key Regulator of Extracellular Matrix and Cell Death in Intervertebral Disc Degeneration. Trentini A, editor. Mediators of Inflammation, 2024, 4482642. https://doi.org/10.1155/2024/4482642
[2] Pizzorno, J. (2014) Glutathione! Integrative Medicine (Encinitas, Calif), 13, 8–12.
[3] Calabrese, G., Morgan, B. and Riemer, J. (2017) Mitochondrial Glutathione: Regulation and Functions. Antioxidants & Redox Signaling, 27, 1162–77. https://doi.org/10.1089/ars.2017.7121
[4] Deponte, M. (2013) Glutathione catalysis and the reaction mechanisms of glutathione-dependent enzymes. Biochimica et Biophysica Acta (BBA) - General Subjects, 1830, 3217–66. https://doi.org/10.1016/j.bbagen.2012.09.018
[5] Yoo, H.C., Yu, Y.C., Sung, Y. and Han, J.M. (2020) Glutamine reliance in cell metabolism. Experimental & Molecular Medicine, 52, 1496–516. https://doi.org/10.1038/s12276-020-00504-8
[6] DeLeve, L.D. and Kaplowitz, N. (1991) Glutathione metabolism and its role in hepatotoxicity. Pharmacology & Therapeutics, 52, 287–305. https://doi.org/10.1016/0163-7258(91)90029-L
[7] Lauterburg, B.H., Adams, J.D. and Mitchell, J.R. (1984) Hepatic Glutathione Homeostasis in the Rat: Efflux Accounts for Glutathione Turnover. Hepatology, 4, 586–90. https://doi.org/10.1002/hep.1840040402
[8] Ookhtens, M., Mittur, A.V. and Erhart, N.A. (1994) Changes in plasma glutathione concentrations, turnover, and disposal in developing rats. American Journal of Physiology-Regulatory, Integrative and Comparative Physiology, 266, R979–88. https://doi.org/10.1152/ajpregu.1994.266.3.R979
[9] Welbourne, T.C. (1979) Ammonia production and glutamine incorporation into glutathione in the functioning rat kidney. Canadian Journal of Biochemistry, 57, 233–7. https://doi.org/10.1139/o79-029
[10] Sappington, D.R., Siegel, E.R., Hiatt, G., Desai, A., Penney, R.B., Jamshidi-Parsian, A. et al. (2016) Glutamine drives glutathione synthesis and contributes to radiation sensitivity of A549 and H460 lung cancer cell lines. Biochimica et Biophysica Acta (BBA) - General Subjects, 1860, 836–43. https://doi.org/10.1016/j.bbagen.2016.01.021
[11] Tompkins, S.C., Sheldon, R.D., Rauckhorst, A.J., Noterman, M.F., Solst, S.R., Buchanan, J.L. et al. (2019) Disrupting Mitochondrial Pyruvate Uptake Directs Glutamine into the TCA Cycle away from Glutathione Synthesis and Impairs Hepatocellular Tumorigenesis. Cell Reports, 28, 2608-2619.e6. https://doi.org/10.1016/j.celrep.2019.07.098
[12] Muir, A., Danai, L.V., Gui, D.Y., Waingarten, C.Y., Lewis, C.A. and Vander Heiden, M.G. (2017) Environmental cystine drives glutamine anaplerosis and sensitizes cancer cells to glutaminase inhibition. eLife, 6, e27713. https://doi.org/10.7554/eLife.27713
[13] Timmerman, L.A., Holton, T., Yuneva, M., Louie, R.J., Padró, M., Daemen, A. et al. (2013) Glutamine Sensitivity Analysis Identifies the xCT Antiporter as a Common Triple-Negative Breast Tumor Therapeutic Target. Cancer Cell, 24, 450–65. https://doi.org/10.1016/j.ccr.2013.08.020
[14] Bachhawat, A.K. and Yadav, S. (2018) The glutathione cycle: Glutathione metabolism beyond the γ‐glutamyl cycle. IUBMB Life, 70, 585–92. https://doi.org/10.1002/iub.1756
[15] Lu, S.C. (2009) Regulation of glutathione synthesis. Molecular Aspects of Medicine, 30, 42–59. https://doi.org/10.1016/j.mam.2008.05.005
[16] Minich, D.M. and Brown, B.I. (2019) A Review of Dietary (Phyto)Nutrients for Glutathione Support. Nutrients, 11, 2073. https://doi.org/10.3390/nu11092073
[17] Marí, M., Morales, A., Colell, A., García-Ruiz, C. and Fernández-Checa, J.C. (2009) Mitochondrial Glutathione, a Key Survival Antioxidant. Antioxidants & Redox Signaling, 11, 2685–700. https://doi.org/10.1089/ars.2009.2695
[18] Filomeni, G., Aquilano, K., Civitareale, P., Rotilio, G. and Ciriolo, M.R. (2005) Activation of c-Jun-N-terminal kinase is required for apoptosis triggered by glutathione disulfide in neuroblastoma cells. Free Radical Biology and Medicine, 39, 345–54. https://doi.org/10.1016/j.freeradbiomed.2005.03.022
[19] Pizzorno, J. (2015) Clinical experience in decreasing mercury load. Integrative Medicine (Encinitas, Calif), 14, 8–13.
[20] Pizzorno, J. (2012) What Should We Tell Our Patients About Alcohol? Integrative Medicine (Encinitas, Calif), 11, 8–11.
[21] Traverso, N., Ricciarelli, R., Nitti, M., Marengo, B., Furfaro, A.L., Pronzato, M.A. et al. (2013) Role of Glutathione in Cancer Progression and Chemoresistance. Oxidative Medicine and Cellular Longevity, 2013, 1–10. https://doi.org/10.1155/2013/972913
[22] Suzuki, H. (1999) Analysis of Xenobiotic Detoxification System Mediated by Efflux Transporters. YAKUGAKU ZASSHI, 119, 822–34. https://doi.org/10.1248/yakushi1947.119.11_822
[23] Shan, X., Aw, T.Y. and Jones, D.P. (1990) Glutathione-dependent projection against oxidative injury. Pharmacology & Therapeutics, 47, 61–71. https://doi.org/10.1016/0163-7258(90)90045-4
[24] Dobashi, K., Aihara, M., Araki, T., Shimizu, Y., Utsugi, M., Iizuka, K. et al. (2002) Regulation of LPS induced IL-12 production by IFN- γ and IL-4 through intracellular glutathione status in human alveolar macrophages. Clinical and Experimental Immunology, 124, 290–6. https://doi.org/10.1046/j.1365-2249.2001.01535.x
[25] Bagherpour, S. and Pérez-García, L. (2024) Recent advances on nanomaterial-based glutathione sensors. Journal of Materials Chemistry B, 12, 8285–309. https://doi.org/10.1039/D4TB01114G
[26] Saharan, S. and Mandal, P.K. (2014) The Emerging Role of Glutathione in Alzheimer’s Disease. Journal of Alzheimer’s Disease, 40, 519–29. https://doi.org/10.3233/JAD-132483
[27] Smeyne, M. and Smeyne, R.J. (2013) Glutathione metabolism and Parkinson’s disease. Free Radical Biology and Medicine, 62, 13–25. https://doi.org/10.1016/j.freeradbiomed.2013.05.001
[28] Frye, R.E. and James, S.J. (2014) Metabolic Pathology of Autism in Relation to Redox Metabolism. Biomarkers in Medicine, 8, 321–30. https://doi.org/10.2217/bmm.13.158
[29] Chauhan, A., Audhya, T. and Chauhan, V. (2012) Brain Region-Specific Glutathione Redox Imbalance in Autism. Neurochemical Research, 37, 1681–9. https://doi.org/10.1007/s11064-012-0775-4
[30] Rosa, A.R., Singh, N., Whitaker, E., De Brito, M., Lewis, A.M., Vieta, E. et al. (2014) Altered plasma glutathione levels in bipolar disorder indicates higher oxidative stress; a possible risk factor for illness onset despite normal brain-derived neurotrophic factor (BDNF) levels. Psychological Medicine, 44, 2409–18. https://doi.org/10.1017/S0033291714000014
[31] Gysin, R., Kraftsik, R., Sandell, J., Bovet, P., Chappuis, C., Conus, P. et al. (2007) Impaired glutathione synthesis in schizophrenia: Convergent genetic and functional evidence. Proceedings of the National Academy of Sciences, 104, 16621–6. https://doi.org/10.1073/pnas.0706778104
[32] Weiduschat, N., Mao, X., Hupf, J., Armstrong, N., Kang, G., Lange, D.J. et al. (2014) Motor cortex glutathione deficit in ALS measured in vivo with the J-editing technique. Neuroscience Letters, 570, 102–7. https://doi.org/10.1016/j.neulet.2014.04.020
[33] Ferreira, B., Mendes, F., Osorio, N., Caseiro, A., Gabriel, A. and Valado, A. (2013) Glutathione in multiple sclerosis. British Journal of Biomedical Science, 70, 75–9. https://doi.org/10.1080/09674845.2013.11669939
[34] Julius, M., Lang, C.A., Gleiberman, L., Harburg, E., Difranceisco, W. and Schork, A. (1994) Glutathione and morbidity in a community-based sample of elderly. Journal of Clinical Epidemiology, 47, 1021–6. https://doi.org/10.1016/0895-4356(94)90117-1
[35] Monti, D.A., Zabrecky, G., Kremens, D., Liang, T., Wintering, N.A., Bazzan, A.J. et al. (2019) N‐Acetyl Cysteine Is Associated With Dopaminergic Improvement in Parkinson’s Disease. Clinical Pharmacology & Therapeutics, 106, 884–90. https://doi.org/10.1002/cpt.1548
[36] Jayaram, L., King, P.T., Hunt, J., Lim, M., Park, C., Hu, E. et al. (2024) Evaluation of high dose N- Acetylcysteine on airway inflammation and quality of life outcomes in adults with bronchiectasis: A randomised placebo-controlled pilot study. Pulmonary Pharmacology & Therapeutics, 84, 102283. https://doi.org/10.1016/j.pupt.2023.102283
[37] Babu Balagopal, P., Kohli, R., Uppal, V., Averill, L., Shah, C., McGoogan, K. et al. (2024) Effect of N ‐acetyl cysteine in children with metabolic dysfunction‐associated steatotic liver disease—A pilot study. Journal of Pediatric Gastroenterology and Nutrition, 79, 652–60. https://doi.org/10.1002/jpn3.12312
[38] Medved, I., Brown, M.J., Bjorksten, A.R., Murphy, K.T., Petersen, A.C., Sostaric, S. et al. (2004) N -acetylcysteine enhances muscle cysteine and glutathione availability and attenuates fatigue during prolonged exercise in endurance-trained individuals. Journal of Applied Physiology, 97, 1477–85. https://doi.org/10.1152/japplphysiol.00371.2004
[39] Amirato, G.R., Borges, J.O., Marques, D.L., Santos, J.M.B., Santos, C.A.F., Andrade, M.S. et al. (2021) L-Glutamine Supplementation Enhances Strength and Power of Knee Muscles and Improves Glycemia Control and Plasma Redox Balance in Exercising Elderly Women. Nutrients, 13, 1025. https://doi.org/10.3390/nu13031025
[40] Kasperczyk, S., Dobrakowski, M., Kasperczyk, A., Romuk, E., Rykaczewska-Czerwińska, M., Pawlas, N. et al. (2016) Effect of N -acetylcysteine administration on homocysteine level, oxidative damage to proteins, and levels of iron (Fe) and Fe-related proteins in lead-exposed workers. Toxicology and Industrial Health, 32, 1607–18. https://doi.org/10.1177/0748233715571152
[41] Almeida, E.B., Santos, J.M.B., Paixão, V., Amaral, J.B., Foster, R., Sperandio, A. et al. (2020) L-Glutamine Supplementation Improves the Benefits of Combined-Exercise Training on Oral Redox Balance and Inflammatory Status in Elderly Individuals. Oxidative Medicine and Cellular Longevity, 2020, 1–13. https://doi.org/10.1155/2020/2852181
[42] Kumar, P., Liu, C., Suliburk, J., Hsu, J.W., Muthupillai, R., Jahoor, F. et al. (2023) Supplementing Glycine and N-Acetylcysteine (GlyNAC) in Older Adults Improves Glutathione Deficiency, Oxidative Stress, Mitochondrial Dysfunction, Inflammation, Physical Function, and Aging Hallmarks: A Randomized Clinical Trial. Lipsitz LA, editor. The Journals of Gerontology: Series A, 78, 75–89. https://doi.org/10.1093/gerona/glac135
Comments (0)
There are no comments for this article. Be the first one to leave a message!