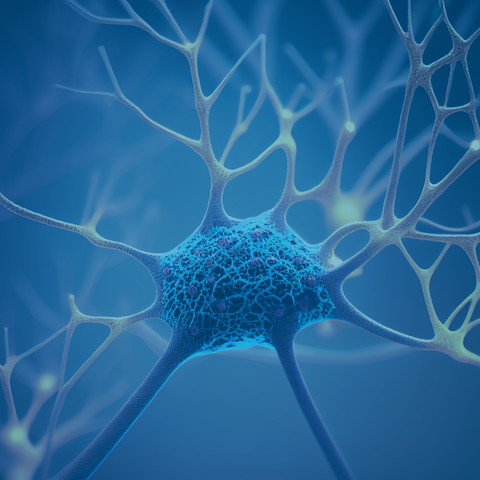
What happens when we put our hand on a hot pan and take it off immediately? The nervous system acts as a control center for our body, receiving signals from sensory neurons and transmitting signals to the motor neurons to control movements and reactions.
The nervous system is the body's electrical wiring system that consists of the brain, spinal cord, and a network of nerves that reach every part of the body. Neurons are structural and functional units of the nervous system. Dendrites are the branching extensions of a neuron that receive signals from other neurons and transmit them to the cell body [4,5]. Dendrites derive their name from the Greek word for “tree,” as they are branching extensions of nerve cells that transmit electrical and chemical signals from other neurons to the neuron's cell body [5].
How are dendrites formed?
The development and growth of dendrites are vital steps in creating neuronal connections. The process of dendrite development and formation is called dendritogenesis. Dendritogenesis is regulated and impacted by the following factors:
1. Protein Kinases
Numerous kinases play a vital role in dendritogenesis. Most importantly, calcium calmodulin-dependent protein kinases (CaMKII), phosphoinositide-3-kinase (PI3K), hippo kinases, and liver kinase B are important players [6,7]. They impact every step of dendritogenesis, including neuronal polarization, spinogenesis, arborization of dendrites, and self-avoidance of dendrites belonging to the same neuron [6].
2. Transcription Factors
Many transcription factors and regulators play an active role in dendritogenesis [4,7,8]. The most important transcription factors are the calcium-responsive transactivator (CREST) and cAMP response binding protein (CREB) [8]. CREST and CREB are activated by calcium signaling and regulate neuronal survival, dendritic growth, synaptic function, and dendrite morphogenesis [7,8].
3. Neurotrophins
Neurotrophins are growth-promoting proteins that are released and play a crucial role in the development of dendrites in the nervous system [4,7]. They play a vital role in the arborization of dendrites and the morphology of the spine [4]. Nerve growth factors (NGF), brain-derived growth factor (BDNF), and neurotrophins 3 and 4 (NT-3 and NT-4) are the four neurotrophins.
Functions of Dendrites
1. Long-term memory
Dendrites are associated with long-term memory (LTM) [9]. Studies have found that in LTM, there is an increase in dendritic spine number and new synapses [9-11]. Eric Kandel elucidated the LTM retention mechanism and was awarded the Nobel Prize in 2000.
2. Motor activities
Dendrites are key in all motor neuron-related functions [12]. Their impairment leads to loss of speech, walking, and visualization.
Diseases associated with dendritogenesis failure
Dendrites play the most critical role in transferring signals from one neuron to another. A failed or abnormal dendritogenesis leads to many neurological disorders [6,12].
1. CDKL5 Syndrome
CDKL5 Syndrome is a rare genetic condition that affects the X chromosome and leads to significant neurodevelopmental problems, including early-onset seizures and intellectual disabilities [6]. The cause of CDKL5 Syndrome is traced to mutations in the CDKL5 gene, which encodes a serine/threonine kinase [6]. Studies found that CDKL5 gene knockout reduces the size of dendrites in the granule cells of the hippocampus.
2. Fragile X Syndrome
Fragile X syndrome (FXS) is the most prevalent hereditary intellectual disability [13]. Studies have observed a significantly higher number of dendritic spines with immature morphology present in FXS patients [12]. The increased number of long dendritic spines and a reduced number of shorter dendritic spines are present in the temporal and visual cortex of patients suffering from FXS compared to the control [12,14,15].
3. Rett Syndrome
Rett Syndrome is a rare neurological disorder commonly found in childhood, especially in girls [16]. It is caused by a rare X-linked mutation in the methyl-CpG-binding protein- 2 (MECP2) gene [16,17]. MECP2 is an essential protein in normal brain development. The lack of normal brain development also causes reduced arborization of dendrites and increased spine dysgenesis. Lower spine density and a decreased number of mushroom-type spines in the cortex and hippocampus are observed in the brain slices of Rett Syndrome patients [12,16,17]. The lack of dendritic spines leads to a range of motor to intellectual deficits. The clinical symptoms include difficulty breathing, speaking, chewing, feeding, and walking.
4. Depression
In modern times, depression impacts roughly 20% of the American population [18]. It is a severe psychiatric disorder characterized by its symptoms, including excessive sleepiness or insomnia, excessive hunger, anxiety, apathy, fatigue, mood swings, grieving, loss of appetite, irritability, agitation, lack of concentration, slowness in activity, and suicidal thoughts [12]. Depression is associated with impairment in dendritic spine morphology and spine density. Studies have found that depression is characterized by reduced dendritic spine number and destabilization of existing dendritic spines [6,12]. These changes lead to decreases in synapses overall and weakened synaptic connectivity.
5. Sleep Disorders
Sleeping is an essential process for the nervous system that significantly impacts the development, preservation, and adaptability of synapses. This is because it is well-known that significant synaptogenesis (read more about the topic here) and cleaning of toxic materials from the brain (read more about the glymphatic system here) happens during sleep [19,20]. Studies have revealed that a lack of sleep or sleep deprivation has harmful effects on dendritic spine communication, forming actin polymer chains, and leads to fluctuations in the shape of dendritic spines [21-23].
6. Neurodegenerative disorders
Impairment in dendritogenesis is a notable feature of neurodegenerative disorders [4,12,24]. In Alzheimer’s disease, there is increased spine loss, premature dendritic spines, and varicosities formation [6]. Decrease in dendritic length, dendritic spine loss, and varicosities formation are observed in Parkinson’s disease [12,25]. Reduction in dendritic arborization, focal swellings on dendrites, and decreased spine density are reported in advanced stages of Huntington's disease [12,26]. In autism, there is a higher dendritic spine density. Dendritic spines show larger spine heads along with a distinct reduction in dendritic spine number and altered spine morphology [6,7]
7. Neurodevelopmental disorders
One of the major causes of neurodevelopmental disorders is the alteration in dendritogenesis [6,12,24,27]. Down’s syndrome is characterized by a reduction in the dendritic spines, arborization of dendrites, and altered spine morphology. Reduction in the development of basal dendrites and higher dendritic spine density with the immature spine is characteristic of autism spectrum disorders [6,24,27].
Dendritogenesis aberration is also associated with diseases like schizophrenia, prion disease, epilepsy, stroke, and mental retardation [12].
Ways to Enhance Dendritogenesis
Dendrites are the most essential part of synaptic formation; hence, forming new dendrites is critical for healthy brain functioning. Here are some ways to enhance it:
1. Meditation
Chronic stress is one of the main causative agents of depression [18]. Scientists have shown that there is an adverse impact on the shape, activity, and function of dendrites, as well as on synaptic plasticity and the secretion of stress hormones (cortisol) due to chronic stress [7,12]. It is crucial to prevent chronic stress to preserve optimal neural and synaptic functioning. Studies have shown many positive effects of meditation on reducing chronic stress [28,29].
2. Sleep
Studies have shown that sleep after motor learning activates the dendritogenesis and spinogenesis of postsynaptic dendrites [21,22]. Sleep also prevents the elimination of newly formed spines after learning multiple tasks [21,22]. Non-rapid eye movement sleep reactivates neurons that are activated during motor tasks. Altering this hinders branch-specific dendritic spine formation [21,22].
3. Antioxidants
Studies have found that a diet rich in antioxidants reduces dendritic spine loss [31]. Antioxidants eliminate reactive oxygen species (ROS) and protect the brain. Omega fatty acids have been shown to improve neuronal function mediated by the formation of new neurons and synapses [31,32]. Omega fatty acids also increase the secretion of neurotrophins like BDNF, NGF, NT-3, and NT-4, activating dendritogenesis [12,33,34].
4. Methylene Blue
Methylene blue has been shown to activate the expression of PTEN-induced kinase 1 (PINK1) [35,36]. PINK1 is actively involved in regulating dendritic morphology [37]. Thus, the application of methylene blue may positively impact dendritogenesis [38]. Methylene blue also activates BDNF [39,40], which has been shown to play an active role in dendritogenesis. Methylene blue enhances antioxidants in the body, which reduces ROS and improves cognitive function [35,38]. You can grab some here.
5. Neurobics
Neurobics (neuron + aerobics) is a concept introduced by Dr. Lawrence Katz [41]. He developed a variety of brain exercises that can provide more oxygen to neurons and help improve cognitive function [41]. The concept is that brain exercises result in a higher release of neurotrophins like BDNF [41-43]. BDNF activates dendritogenesis and helps in improving cognitive function [42,43]. Practicing long-term neurobics may induce a change in brain morphology due to neuroplasticity. Studies have found that London taxi drivers have increased grey matter and larger hippocampal volume, as driving is a neurobic that impacts these brain regions [42,44]. Similarly, learning music improves verbal and working memory [41,42].
6. Physical Exercise
Regular aerobic exercise enhances neurogenesis, synaptic signaling, and dendritic spine number in elderly people [12]. Daily exercise protects neuronal and synaptic loss and delays the onset or progression [45,46].
Conclusion
Dendrites are a vital part of the neuronal system and are involved in the development of many neuro-related disorders.
Treatments that aim to restore dendrites and their spines can enhance motor abilities and overall quality of life as well as slow down the advancement and onset of degenerative symptoms.
Further studies are underway and examining dendrites on various levels, including their shape, function, biochemistry, and molecular makeup. It is crucial to comprehend the cellular processes and signaling pathways involved in dendritogenesis and how they affect neural networks' structural adaptability during healthy and diseased states. This understanding is essential to grasping the role of dendrites in cognition and behavior.
How are you enhancing dendritogenesis today? Let us know in the comments!
References
- Kandel, E.R.; Dudai, Y.; Mayford, M.R. The Molecular and Systems Biology of Memory. Cell 2014, 157, 163–186.
- Südhof, T.C. The Molecular Machinery of Neurotransmitter Release (Nobel Lecture). Angewandte Chemie - International Edition 2014, 53, 12696–12717, doi:10.1002/anie.201406359.
- Südhof, T.C. Towards an Understanding of Synapse Formation. Neuron 2018, 100, 276–293, doi:10.1016/j.neuron.2018.09.040.
- Puram, S. v.; Bonni, A. Cell-Intrinsic Drivers of Dendrite Morphogenesis. Development (Cambridge) 2013, 140, 4657–4671.
- DeFelipe, J. The Dendritic Spine Story: An Intriguing Process of Discovery. Front Neuroanat 2015, 9.
- Nourbakhsh, K.; Yadav, S. Kinase Signaling in Dendritic Development and Disease. Front Cell Neurosci 2021, 15.
- Valnegri, P.; Puram, S. v.; Bonni, A. Regulation of Dendrite Morphogenesis by Extrinsic Cues. Trends Neurosci 2015, 38, 439–447.
- de la Torre-Ubieta, L.; Bonni, A. Transcriptional Regulation of Neuronal Polarity and Morphogenesis in the Mammalian Brain. Neuron 2011, 72, 22–40.
- Hongpaisan, J.; Alkon, D.L. A Structural Basis for Enhancement of Long-Term Associative Memory in Single Dendritic Spines Regulated by PKC. Proceedings of the National Academy of Sciences 2007, 104, 19571–19576.
- Sheffield, M.E.J.; Dombeck, D.A. Calcium Transient Prevalence across the Dendritic Arbour Predicts Place Field Properties. Nature 2015, 517, 200–204.
- Basu, S.; Lamprecht, R. The Role of Actin Cytoskeleton in Dendritic Spines in the Maintenance of Long-Term Memory. Front Mol Neurosci 2018, 11, 143.
- Chidambaram, S.B.; Rathipriya, A.G.; Bolla, S.R.; Bhat, A.; Ray, B.; Mahalakshmi, A.M.; Manivasagam, T.; Thenmozhi, A.J.; Essa, M.M.; Guillemin, G.J.; et al. Dendritic Spines: Revisiting the Physiological Role. Prog Neuropsychopharmacol Biol Psychiatry 2019, 92, 161–193.
- Hall, S.S.; Walter, E.; Sherman, E.; Hoeft, F.; Reiss, A.L. The Neural Basis of Auditory Temporal Discrimination in Girls with Fragile X Syndrome. J Neurodev Disord 2009, 1, 91–99.
- Walter, E.; Mazaika, P.K.; Reiss, A.L. Insights into Brain Development from Neurogenetic Syndromes: Evidence from Fragile X Syndrome, Williams Syndrome, Turner Syndrome and Velocardiofacial Syndrome. Neuroscience 2009, 164, 257–271.
- Cruz-Martín, A.; Crespo, M.; Portera-Cailliau, C. Delayed Stabilization of Dendritic Spines in Fragile X Mice. Journal of Neuroscience 2010, 30, 7793–7803.
- Chahrour, M.; Zoghbi, H.Y. The Story of Rett Syndrome: From Clinic to Neurobiology. Neuron 2007, 56, 422–437.
- Chapleau, C.A.; Calfa, G.D.; Lane, M.C.; Albertson, A.J.; Larimore, J.L.; Kudo, S.; Armstrong, D.L.; Percy, A.K.; Pozzo-Miller, L. Dendritic Spine Pathologies in Hippocampal Pyramidal Neurons from Rett Syndrome Brain and after Expression of Rett-Associated MECP2 Mutations. Neurobiol Dis 2009, 35, 219–233.
- Schlosser, R.J.; Gage, S.E.; Kohli, P.; Soler, Z.M. Burden of Illness: A Systematic Review of Depression in Chronic Rhinosinusitis. Am J Rhinol Allergy 2016, 30, 250–256.
- Hauglund, N.L.; Pavan, C.; Nedergaard, M. Cleaning the Sleeping Brain–the Potential Restorative Function of the Glymphatic System. Curr Opin Physiol 2020, 15, 1–6.
- Petzoldt, A.G.; Sigrist, S.J. Synaptogenesis. Current Biology 2014, 24, R1076–R1080, doi:10.1016/j.cub.2014.10.024.
- Yang, G.; Lai, C.S.W.; Cichon, J.; Ma, L.; Li, W.; Gan, W.-B. Sleep Promotes Branch-Specific Formation of Dendritic Spines after Learning. Science (1979) 2014, 344, 1173–1178.
- Yang, G.; Gan, W. Sleep Contributes to Dendritic Spine Formation and Elimination in the Developing Mouse Somatosensory Cortex. Dev Neurobiol 2012, 72, 1391–1398.
- Ribeiro, S.; Gervasoni, D.; Soares, E.S.; Zhou, Y.; Lin, S.-C.; Pantoja, J.; Lavine, M.; Nicolelis, M.A.L. Long-Lasting Novelty-Induced Neuronal Reverberation during Slow-Wave Sleep in Multiple Forebrain Areas. PLoS Biol 2004, 2, e24.
- Copf, T. Impairments in Dendrite Morphogenesis as Etiology for Neurodevelopmental Disorders and Implications for Therapeutic Treatments. Neurosci Biobehav Rev 2016, 68, 946–978.
- Villalba, R.M.; Smith, Y. Differential Striatal Spine Pathology in Parkinson’s Disease and Cocaine Addiction: A Key Role of Dopamine? Neuroscience 2013, 251, 2–20.
- Ferrante, R.J.; Kowall, N.W.; Richardson, E.P. Proliferative and Degenerative Changes in Striatal Spiny Neurons in Huntington’s Disease: A Combined Study Using the Section-Golgi Method and Calbindin D28k Immunocytochemistry. Journal of Neuroscience 1991, 11, 3877–3887.
- Wayman, G.A.; Lee, Y.S.; Tokumitsu, H.; Silva, A.; Soderling, T.R. Calmodulin-Kinases: Modulators of Neuronal Development and Plasticity. Neuron 2008, 59, 914–931.
- Green, A.A.; Kinchen, E. v The Effects of Mindfulness Meditation on Stress and Burnout in Nurses. Journal of Holistic Nursing 2021, 39, 356–368.
- Dunlop, J. Meditation, Stress Relief, and Well-Being. Radiol Technol 2015, 86, 535–555.
- Ji, D.; Wilson, M.A. Coordinated Memory Replay in the Visual Cortex and Hippocampus during Sleep. Nat Neurosci 2007, 10, 100–107.
- Johnson, J.B.; Summer, W.; Cutler, R.G.; Martin, B.; Hyun, D.-H.; Dixit, V.D.; Pearson, M.; Nassar, M.; Tellejohan, R.; Maudsley, S. Alternate Day Calorie Restriction Improves Clinical Findings and Reduces Markers of Oxidative Stress and Inflammation in Overweight Adults with Moderate Asthma. Free Radic Biol Med 2007, 42, 665–674.
- Colman, R.J.; Anderson, R.M.; Johnson, S.C.; Kastman, E.K.; Kosmatka, K.J.; Beasley, T.M.; Allison, D.B.; Cruzen, C.; Simmons, H.A.; Kemnitz, J.W. Caloric Restriction Delays Disease Onset and Mortality in Rhesus Monkeys. Science (1979) 2009, 325, 201–204.
- Wu, A.; Ying, Z.H.E.; Gomez-Pinilla, F. Omega-3 Fatty Acids Supplementation Restores Mechanisms That Maintain Brain Homeostasis in Traumatic Brain Injury. J Neurotrauma 2007, 24, 1587–1595.
- Gómez-Pinilla, F. Brain Foods: The Effects of Nutrients on Brain Function. Nat Rev Neurosci 2008, 9, 568–578.
- Yang, L.; Youngblood, H.; Wu, C.; Zhang, Q. Mitochondria as a Target for Neuroprotection: Role of Methylene Blue and Photobiomodulation. Transl Neurodegener 2020, 9, 1–22.
- Murata, H.; Takamatsu, H.; Liu, S.; Kataoka, K.; Huh, N.; Sakaguchi, M. NRF2 Regulates PINK1 Expression under Oxidative Stress Conditions. PLoS One 2015, 10, e0142438.
- das Banerjee, T.; Dagda, R.Y.; Dagda, M.; Chu, C.T.; Rice, M.; Vazquez‐Mayorga, E.; Dagda, R.K. PINK 1 Regulates Mitochondrial Trafficking in Dendrites of Cortical Neurons through Mitochondrial PKA. J Neurochem 2017, 142, 545–559.
- Krutskikh, E.P.; Potanina, D. v.; Samoilova, N.A.; Gryaznova, M. v.; Sadovnikova, I.S.; Gureev, A.P.; Popov, V.N. Brain Protection by Methylene Blue and Its Derivative, Azur B, via Activation of the Nrf2/ARE Pathway in Cisplatin-Induced Cognitive Impairment. Pharmaceuticals 2022, 15.
- Ahmed, M.E.; Tucker, D.; Dong, Y.; Lu, Y.; Zhao, N.; Wang, R.; Zhang, Q. Methylene Blue Promotes Cortical Neurogenesis and Ameliorates Behavioral Deficit after Photothrombotic Stroke in Rats. Neuroscience 2016, 336, 39–48, doi:10.1016/j.neuroscience.2016.08.036.
- Bhurtel, S.; Katila, N.; Neupane, S.; Srivastav, S.; Park, P.; Choi, D. Methylene Blue Protects Dopaminergic Neurons against MPTP‐induced Neurotoxicity by Upregulating Brain‐derived Neurotrophic Factor. Ann N Y Acad Sci 2018, 1431, 58–71.
- Katz, L. Keep Your Brain Alive: 83 Neurobic Exercises to Help Prevent Memory Loss and Increase Mental Fitness; Workman Publishing, 2014; ISBN 0761168931.
- Castillo, M. Boosting Your Brain, Part 1: The Couch Potato. American Journal of Neuroradiology 2013, 34, 693–695.
- Serbin, K.P. BDNF and ‘Neurobics’: Building a ‘Beautiful Mind’against Huntington’s Available online: https://digital.sandiego.edu/huntingtons/107 (accessed on 12 February 2023).
- Maguire, E.A.; Woollett, K.; Spiers, H.J. London Taxi Drivers and Bus Drivers: A Structural MRI and Neuropsychological Analysis. Hippocampus 2006, 16, 1091–1101.
- Ghalandari-Shamami, M.; Nourizade, S.; Yousefi, B.; Vafaei, A.A.; Pakdel, R.; Rashidy-Pour, A. Beneficial Effects of Physical Activity and Crocin against Adolescent Stress Induced Anxiety or Depressive-like Symptoms and Dendritic Morphology Remodeling in Prefrontal Cortex in Adult Male Rats. Neurochem Res 2019, 44, 917–929.
- Wang, P.; Liang, Y.; Chen, K.; Yau, S.-Y.; Sun, X.; Cheng, K.K.-Y.; Xu, A.; So, K.-F.; Li, A. Potential Involvement of Adiponectin Signaling in Regulating Physical Exercise-Elicited Hippocampal Neurogenesis and Dendritic Morphology in Stressed Mice. Front Cell Neurosci 2020, 14, 189.
Comments (0)
There are no comments for this article. Be the first one to leave a message!