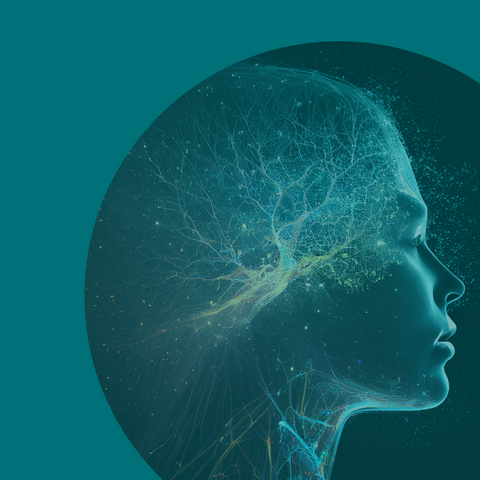
Have you ever wondered how your body clears cellular waste? Where do our millions of cells dump their garbage?
The answer to this question is relatively simple: outside of your brain, all of the cells in your body pour their harmful metabolic waste, proteins, and other dissolved substances into the lymphatic system. Just like the sewer pipes in a house carry waste to the septic tank, here inside the body, lymphatic vessels carry, and then dispose of, waste material [1,2].
During the 20th century, it was believed that waste clearance of the brain and spinal cord was carried out by the movement of cerebrospinal fluid (CSF). The CSF served as a "sink" and eliminated waste from the brain [3,4], but exactly how this worked was unclear.
Due to advances in technology (specifically imaging), the glymphatic system (which stands for glial-dependent lymphatic transport) was discovered in 2012 [3].
Let's take a deep dive into the glymphatic system. We'll discuss its discovery, its mechanisms of action, ways to modulate it, and diseases associated with poor glymphatic flow.
A Brief History of the Glymphatic System
The first evidence of brain waste clearance was observed by Italian anatomist Paolo Mascagni (1755-1815), who had already depicted meningeal lymphatic vessels in a hand-drawn illustration almost 200 years ago [5]. The Cserr group first documented the existence of convective clearance in 1981 [6], and a decade later, the Rennels group showed that CSF is quickly transported along perivascular spaces [7].
With advanced technology, a group of researchers led by Dr. Maiken Nedergaard at the University of Rochester Medical Center in 2012 showed that waste clearance in the brain is similar to that of the lymphatic system [3,8]. The waste clearance system in the brain was then named the glymphatic system for glial-dependent lymphatic transport.
The glymphatic system was responsible for removing soluble proteins and metabolites from the central nervous system, including β-amyloid and τ-proteins, which are associated with neurodegenerative disorders, although there is recent controversy around these long-purported correlations [2,3].
Why did it take so long to discover the glymphatic system?
We've known about many cellular components of the brain for decades if not centuries. For example, the understanding of synapses originated in the late 18th century and this research led to several Nobel Prizes [9] (see our article on synaptogenesis here).
One of the key reasons the glymphatic system evaded the scientific community was that, as opposed to synaptic and other discoveries, it could not be detected in brain tissue samples [2]. With the advancement in imaging technology, however, (namely two-photon microscopy) scientists were able to discover the plumbing system responsible for flushing waste throughout the brain [3].
Discovery of the Glymphatic System (Early Mice Studies)
Researchers focused on the movement of CSF in the brains of mice, illustrating its direction and speed with differently-sized radiolabeled tracers using two-photon imaging techniques [3]. They identified a pathway for fluid transport in the brains of mice that involved the flow of subarachnoid CSF into the brain tissue, followed by the removal of this fluid through large veins. This flow was driven by water movement across astrocytes and was crucial for clearing waste products, including the protein β-amyloid, from the brain [3].
Further studies on the mouse glymphatic system established that the brain performs this function mainly during sleep [10,11]. The discoverer of this system, Dr. Maiken Nedergaard, said, "I think we have discovered why we sleep. We sleep to clean our brain... You can think of it like having a house party. You can either entertain the guests or clean up the house, but you can't do both at the same time" [12].
Constituents of the Glymphatic System
The glymphatic system is composed of specialized glial cells, known as astrocytes, which form a network of channels around blood vessels in the brain [1]. These channels, known as perivascular spaces, are lined with a special type of astrocyte known as a perivascular astrocyte. These astrocytes act as a barrier, preventing the entry of harmful molecules into the brain and also helping to clear waste products from the brain [4]. The glymphatic system is constantly filtering toxins from the brain, but during wakefulness, this system is mainly disengaged. This is why sleep is so important (see below).
3 Drivers of Glymphatic Flow
- Pressure by the generation of CSF: The continuous production of CSF by the choroid plexus generates pressure that determines the fluid flow through the ventricular system into the subarachnoid space [4].
- Respiration: Studies have found that respiration plays an essential role in the movement of CSF [25,26].
- Artery pulsation: Studies show that glymphatic activity is triggered by arterial pulsation [27]. This is why the fluid influx occurs primarily in areas around the pulsating arteries and not the veins in the brain.
Glymphatic System Modulation
Sleep
Several studies have now shown that impairment of sleep is linked to many neurodegenerative disorders [1,2,4,28]. Sleep scientist Matthew Walker once stated, "During sleep, a remarkable sewage system in the brain, called the glymphatic system, kicks into high gear. The brain uses the glymphatic system to clear its waste during sleep. Without sufficient sleep, you fail to get that power to cleanse. With each passing night of insufficient sleep, Alzheimer's Disease risk escalates like compounding interest on a loan [29]." In addition, norepinephrine levels decline during sleep, which leads to an expansion of the brain’s extracellular space. This allows for a decreased resistance to fluid flow. As a result, the glymphatic system is most active in sleep, specifically in N3 or slow-wave sleep which is categorized by slow oscillatory brain waves that create a flux of CSF within the interstitial cavities, leading to an increase in glymphatic clearance [41].
Aging
The glymphatic system efficiency decreases as we age, which could be one of the main reasons aging is associated with neurodegenerative disorders. A study comparing glymphatic function in old and young mice found a significant decrease of 80-90% in aged mice compared to young mice [1,4]. This could at least partially be related to the fact that sleep quality declines as we age [42].
AQP4
The role of the gene AQP4 is well-established in glymphatic system regulation. Even though there are controversies around it, it is well understood that AQP4 is the most critical player. The deletion of AQP4 leads to a 55% reduction in the clearance of β-amyloid, which could lead to neurodegenerative disease [2,4,19]. Although, again, there is some controversy around these studies, which you can read about here.
Sleep Position
Studies have shown that there are ideal sleep positions to maximize the efficiency of the brain's waste-clearance system. Lateral or side body posture while sleeping positively affects the efficiency of the glymphatic system more than sleeping on one's back or stomach [30-32].
Wine (Yes, but...!)
Studies have shown that low levels of wine consumption may improve the function of the glymphatic system, as it reduces inflammation and hence helps to clear waste from the brain [33]. But be careful here, folks, there are many studies showing that even small amounts of regular alcohol intake will also shrink your brain. Sorry!
Diseases associated with glymphatic system dysregulation
Alzheimer's Disease (AD)
Studies have found that good restorative sleep is most important for clearing β-amyloid proteins. As the glymphatic system is actively involved in removing τ- and β-amyloid proteins, malfunctioning or reducing glymphatic function may increase the risk of AD [2,12,28,36]. Researchers observed that CSF could clear the labelled β-amyloid proteins like a dishwasher at double speed when they were injected into the brains of sleeping mice compared to awake mice [2,34].
Parkinson's Disease (PD)
The glymphatic system removes the α-synuclein protein as part of the waste clearance mechanism in the brain. The aggregation of the α-synuclein protein may lead to PD [28]. This aggregation is first spread through the brainstem and the area responsible for the sense of smell, then to the structures involved in emotions and memory, and finally to the outer layer of the brain, which is responsible for higher cognitive functions [28,30]. Sleep impairment is associated with PD, and a new hypothesis suggests that it could be due to reduced activity of the glymphatic system [28,30].
Multiple Sclerosis (MS)
MS is a chronic autoimmune disease that affects the central nervous system. A retrospective study enrolled 71 MS patients to assess the impairment of the glymphatic system [35]. The study found that the glymphatic system was impaired, especially in progressive stages. They also observed that diminished glymphatic function was associated with demyelination and neurodegeneration [35]. These results suggest that the glymphatic system's malfunctioning may play a role in the development of MS. Further research is needed to understand how it interacts with other factors that contribute to the disease [35,36].
Brain Injury
A traumatic brain injury can impact the functioning of the glymphatic system. Studies found that large numbers of τ-proteins drift in the space between the brain's cells during trauma. As the trauma impairs the glymphatic system, accumulating these proteins may cause permanent and progressive damage to brain tissue [28,29].
Cardiovascular Disease
Previous research has shown that the glymphatic system is less functional in hypertensive rodent models [37-39]. Those with cardiovascular disease often have compromised sleep quality, which is possibly connected to poor glymphatic clearance, leading to the build-up of toxic proteins and cognitive decline [38].
Hence, it is not surprising that heart conditions that result in a decrease in blood flow, such as heart failure and irregular heartbeats, would also be linked to a decline in glymphatic flow as the pulsing of the brain's arteries (which drives the glymphatic system) is also reduced [28,38,39]
Small Vessel Disease (SVD)
SVD is a condition that affects the small blood vessels in the brain, in which the walls of the small arteries become thicker over time. Many studies have linked the failure of the glymphatic system to SVD [1,28,30,40].
Summary
Fun fact: In the animal kingdom, humans stand alone as the only species to purposely deprive ourselves of sleep. And when we don't get restorative sleep (see our article on why we sleep, which includes sleep physiology), our glymphatic systems can't operate at full capacity. This, as you know now, is not a good thing... as it gives you a higher risk of almost everything bad happening to your brain.
So when you can, get your beauty rest. It helps to have a dialed-in sleep routine and, if needed, some Tro Calm to quiet your mind and allow yourself to flow merrily down the stream (because life is but a dream)!
References
- Lohela, T.J.; Lilius, T.O.; Nedergaard, M. The Glymphatic System: Implications for Drugs for Central Nervous System Diseases. Nat. Rev. Drug Discov. 2022, 21, 763–779, doi:10.1038/s41573-022-00500-9.
- Nedergaard, M. Garbage Truck of the Brain. Science (80-. ). 2013, 340, 1529–1530, doi:10.1126/science.1240514.
- Iliff, J.J.; Wang, M.; Liao, Y.; Plogg, B.A.; Peng, W.; Gundersen, G.A.; Benveniste, H.; Vates, G.E.; Deane, R.; Goldman, S.A.; et al. A Paravascular Pathway Facilitates CSF Flow through the Brain Parenchyma and the Clearance of Interstitial Solutes, Including Amyloid β. Sci. Transl. Med. 2012, 4, 1–12, doi:10.1126/scitranslmed.3003748.
- Jessen, N.A.; Munk, A.S.F.; Lundgaard, I.; Nedergaard, M. The Glymphatic System: A Beginner’s Guide. Neurochem. Res. 2015, 40, 2583–2599, doi:10.1007/s11064-015-1581-6.
- Sandrone, S.; Moreno-Zambrano, D.; Kipnis, J.; van Gijn, J. A (Delayed) History of the Brain Lymphatic System. Nat. Med. 2019, 25, 538–540.
- Cserr, H.F.; Cooper, D.N.; Suri, P.K.; Patlak, C.S. Efflux of Radiolabeled Polyethylene Glycols and Albumin from Rat Brain. Am. J. Physiol. Physiol. 1981, 240, F319–F328.
- Rennels, M.L.; Blaumanis, O.R.; Grady, P.A. Rapid Solute Transport throughout the Brain via Paravascular Fluid Pathways. Adv. Neurol. 1990, 52, 431–439.
- Begley, D.J. Brain Superhighways. Sci. Transl. Med. 2012, 4, 1–4, doi:10.1126/scitranslmed.3004611.
- Südhof, T.C. The Molecular Machinery of Neurotransmitter Release (Nobel Lecture). Angew. Chemie - Int. Ed. 2014, 53, 12696–12717, doi:10.1002/anie.201406359.
- Hauglund, N.L.; Pavan, C.; Nedergaard, M. Cleaning the Sleeping Brain–the Potential Restorative Function of the Glymphatic System. Curr. Opin. Physiol. 2020, 15, 1–6.
- Lee, H.-J.; Lee, D.A.; Shin, K.J.; Park, K.M. Glymphatic System Dysfunction in Obstructive Sleep Apnea Evidenced by DTI-ALPS. Sleep Med. 2022, 89, 176–181.
- Sample, I. Why Do We Go to Sleep? To Clean Our Brains, Say US Scientists. Guard. 2013.
- Kaur, J.; Davoodi-Bojd, E.; Fahmy, L.M.; Zhang, L.; Ding, G.; Hu, J.; Zhang, Z.; Chopp, M.; Jiang, Q. Magnetic Resonance Imaging and Modeling of the Glymphatic System. Diagnostics 2020, 10, 344.
- Yang, G.; Deng, N.; Liu, Y.; Gu, Y.; Yao, X. Evaluation of Glymphatic System Using Diffusion MR Technique in T2DM Cases. Front. Hum. Neurosci. 2020, 14, 300.
- Taoka, T.; Naganawa, S. Glymphatic Imaging Using MRI. J. Magn. Reson. Imaging 2020, 51, 11–24.
- Eide, P.K.; Ringstad, G. MRI with Intrathecal MRI Gadolinium Contrast Medium Administration: A Possible Method to Assess Glymphatic Function in Human Brain. Acta Radiol. open 2015, 4, 2058460115609635.
- Fultz, N.E.; Bonmassar, G.; Setsompop, K.; Stickgold, R.A.; Rosen, B.R.; Polimeni, J.R.; Lewis, L.D. Coupled Electrophysiological, Hemodynamic, and Cerebrospinal Fluid Oscillations in Human Sleep. Science (80-. ). 2019, 366, 628–631, doi:10.1126/science.aax5440.
- Mestre, H.; Mori, Y.; Nedergaard, M. The Brain’s Glymphatic System: Current Controversies. Trends Neurosci. 2020, 43, 458–466, doi:10.1016/j.tins.2020.04.003.
- Smith, A.J.; Yao, X.; Dix, J.A.; Jin, B.-J.; Verkman, A.S. Test of the’glymphatic’hypothesis Demonstrates Diffusive and Aquaporin-4-Independent Solute Transport in Rodent Brain Parenchyma. Elife 2017, 6, e27679.
- Mestre, H.; Hablitz, L.M.; Xavier, A.L.R.; Feng, W.; Zou, W.; Pu, T.; Monai, H.; Murlidharan, G.; Castellanos Rivera, R.M.; Simon, M.J.; et al. Aquaporin-4-Dependent Glymphatic Solute Transport in the Rodent Brain. Elife 2018, 7, e40070, doi:10.7554/eLife.40070.
- Bedussi, B.; Almasian, M.; de Vos, J.; VanBavel, E.; Bakker, E.N.T.P. Paravascular Spaces at the Brain Surface: Low Resistance Pathways for Cerebrospinal Fluid Flow. J. Cereb. Blood Flow Metab. 2018, 38, 719–726.
- Plog, B.A.; Mestre, H.; Olveda, G.E.; Sweeney, A.M.; Kenney, H.M.; Cove, A.; Dholakia, K.Y.; Tithof, J.; Nevins, T.D.; Lundgaard, I. Transcranial Optical Imaging Reveals a Pathway for Optimizing the Delivery of Immunotherapeutics to the Brain. JCI insight 2018, 3.
- Koundal, S.; Elkin, R.; Nadeem, S.; Xue, Y.; Constantinou, S.; Sanggaard, S.; Liu, X.; Monte, B.; Xu, F.; Van Nostrand, W. Optimal Mass Transport with Lagrangian Workflow Reveals Advective and Diffusion Driven Solute Transport in the Glymphatic System. Sci. Rep. 2020, 10, 1990.
- Jenning, M.; Marklein, B.; Ytterberg, J.; Zubarev, R.A.; Joshua, V.; Van Schaardenburg, D.; Van De Stadt, L.; Catrina, A.I.; Nonhoff, U.; Häupl, T.; et al. Bacterial Citrullinated Epitopes Generated by Porphyromonas Gingivalis Infection-a Missing Link for ACPA Production. Ann. Rheum. Dis. 2020, 79, 1194–1202, doi:10.1136/annrheumdis-2019-216919.
- Yamada, S.; Miyazaki, M.; Yamashita, Y.; Ouyang, C.; Yui, M.; Nakahashi, M.; Shimizu, S.; Aoki, I.; Morohoshi, Y.; McComb, J.G. Influence of Respiration on Cerebrospinal Fluid Movement Using Magnetic Resonance Spin Labeling. Fluids Barriers CNS 2013, 10, 1–7.
- Klose, U.; Strik, C.; Kiefer, C.; Grodd, W. Detection of a Relation between Respiration and CSF Pulsation with an Echoplanar Technique. J. Magn. Reson. Imaging 2000, 11, 438–444.
- Bilston, L.E.; Stoodley, M.A.; Fletcher, D.F. The Influence of the Relative Timing of Arterial and Subarachnoid Space Pulse Waves on Spinal Perivascular Cerebrospinal Fluid Flow as a Possible Factor in Syrinx Development. J. Neurosurg. 2010, 112, 808–813.
- Nedergaard, M.; Goldman, S.A. Glymphatic Failure as a Final Common Pathway to Dementia. Science (80-. ). 2020, 370, 50–56, doi:10.1126/science.abb8739.
- Iliff, J.J.; Chen, M.J.; Plog, B.A.; Zeppenfeld, D.M.; Soltero, M.; Yang, L.; Singh, I.; Deane, R.; Nedergaard, M. Impairment of Glymphatic Pathway Function Promotes Tau Pathology after Traumatic Brain Injury. J. Neurosci. 2014, 34, 16180–16193, doi:10.1523/JNEUROSCI.3020-14.2014.
- Toriello, M.; González-Quintanilla, V.; Pascual, J. The Glymphatic System and Its Involvement in Disorders of the Nervous System. Med. Clin. (Barc). 2021, 156, 339–343, doi:10.1016/j.medcli.2020.08.020.
- Lee, H.; Xie, L.; Yu, M.; Kang, H.; Feng, T.; Deane, R.; Logan, J.; Nedergaard, M.; Benveniste, H. The Effect of Body Posture on Brain Glymphatic Transport. J. Neurosci. 2015, 35, 11034–11044, doi:10.1523/JNEUROSCI.1625-15.2015.
- Benveniste, H.; Liu, X.; Koundal, S.; Sanggaard, S.; Lee, H.; Wardlaw, J. The Glymphatic System and Waste Clearance with Brain Aging: A Review. Gerontology 2019, 65, 106–119, doi:10.1159/000490349.
- Lundgaard, I.; Wang, W.; Eberhardt, A.; Vinitsky, H.S.; Reeves, B.C.; Peng, S.; Lou, N.; Hussain, R.; Nedergaard, M. Beneficial Effects of Low Alcohol Exposure, but Adverse Effects of High Alcohol Intake on Glymphatic Function. Sci. Rep. 2018, 8, 1–16, doi:10.1038/s41598-018-20424-y.
- Xie, L.; Kang, H.; Xu, Q.; Chen, M.J.; Liao, Y.; Thiyagarajan, M.; O’Donnell, J.; Christensen, D.J.; Nicholson, C.; Iliff, J.J.; et al. Sleep Drives Metabolite Clearance from the Adult Brain. Science (80-. ). 2013, 342, 373–377, doi:10.1126/science.1241224.
- Carotenuto, A.; Cacciaguerra, L.; Pagani, E.; Preziosa, P.; Filippi, M.; Rocca, M.A. Glymphatic System Impairment in Multiple Sclerosis: Relation with Brain Damage and Disability. Brain 2022, 145, 2785–2795.
- Scollato, A.; Lolli, F.; Lastrucci, G.; Repice, A.; De Santis, G.; Nicoletti, C.; Porfirio, B.; Gallina, P. Case Report: A Multiple Sclerosis Patient with Imaging Features of Glymphatic Failure Benefitted from CSF Flow Shunting. Front. Neurosci. 2022, 16.
- Mortensen, K.N.; Sanggaard, S.; Mestre, H.; Lee, H.; Kostrikov, S.; Xavier, A.L.R.; Gjedde, A.; Benveniste, H.; Nedergaard, M. Impaired Glymphatic Transport in Spontaneously Hypertensive Rats. J. Neurosci. 2019, 39, 6365–6377.
- Gonzaga, C.; Bertolami, A.; Bertolami, M.; Amodeo, C.; Calhoun, D. Obstructive Sleep Apnea, Hypertension and Cardiovascular Diseases. J. Hum. Hypertens. 2015, 29, 705–712.
- Wendell, C.R.; Gunstad, J.; Waldstein, S.R.; Wright, J.G.; Ferrucci, L.; Zonderman, A.B. Cardiorespiratory Fitness and Accelerated Cognitive Decline with Aging. Journals Gerontol. Ser. A Biomed. Sci. Med. Sci. 2014, 69, 455–462.
- Lv, T.; Zhao, B.; Hu, Q.; Zhang, X. The Glymphatic System: A Novel Therapeutic Target for Stroke Treatment. Front. Aging Neurosci. 2021, 13, 1–16, doi:10.3389/fnagi.2021.689098.
- Lee H., Xie L., Yu M., Kang H., Feng T., Deane R., Benveniste H. The Effect of Body Posture on Brain Glymphatic Transport. J. Neurosci. 2015;35:11034–11044. doi: 10.1523/JNEUROSCI.1625-15.2015. [PMC free article] [PubMed] [CrossRef] [Google Scholar]
- Espiritu JR. Aging-related sleep changes. Clin Geriatr Med. 2008;24(1):1–14, v. [PubMed] [Google Scholar]
Comments (1)
A simple and engaging read.
Helps one to better understand their system.