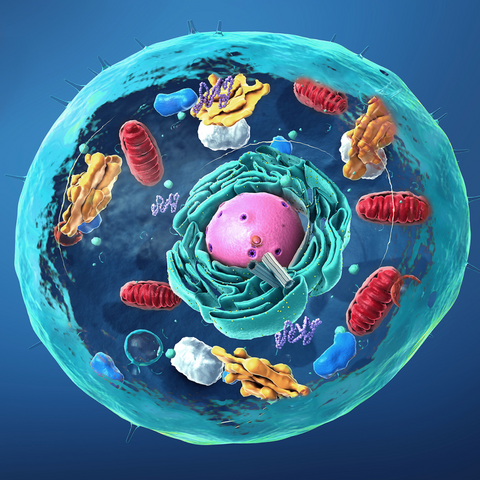
Danger! Watch yourself!
The cell danger response (CDR) may just be the most important cellular response that you've never heard of… until now. Well, unless it was in a Mystical song from the year 2000.
The CDR is an evolutionary conserved metabolic response, activated when a cell comes across serious threats that could potentially injure or kill it [1].
Cells are regularly exposed to harmful threats such as heat shock, ultraviolet radiation, ionizing radiation, toxic chemicals, toxins, or microbes that could potentially kill or injure them. As a result, they have developed complex ways to respond to these dangerous threats, and these mechanisms range from metabolism changes to shutting down critical biological processes that might otherwise be permanently damaged.
Evolutionary History of the Cell Danger Response
Life began on earth 3.5 billion years ago with single-cell organisms and a reducing atmosphere (i.e., no oxygen around!). The atmosphere changed from reducing to oxidizing when the “Great Oxidation Event” happened around 2.5 billion years ago. With ample oxygen available in the atmosphere, organisms reoriented their metabolic strategies and aerobic metabolism started [2]. The coupling of oxygen consumption to ATP synthesis (energy generation) led to the bioenergetics of aerobic bacteria and eukaryotic animals [3]. The evolution from anaerobic to aerobic metabolism also paved the way for the CDR.
Many Names for the Cell Danger Response
In scientific literature, the CDR is known by many names, all of which allude to its main role: to defend the cell. The terminology reflects the confluence of various fields and tools used to study them. These include the heat shock protein response [4], inflammation [5], the ubiquitination stress response [6], innate immunity [7], oxidative stress response [8], oxidative shielding response [9], the mitochondrial unfolded protein response [10], the unfolded protein response [11], the endoplasmic reticulum stress response [11], and integrated stress response [12].
The Cell Danger Response is Evolutionarily Conserved
In aerobic metabolism, reactive oxygen species (ROS) are an inevitable consequence of energy metabolism. The electron transport chain mainly generates ROS in mitochondria, the endoplasmic reticulum, and nuclear membranes. ROS are continuously produced and eliminated to maintain the cells in a steady state. Oxidative stress is triggered in a cell when the cellular environment becomes highly oxidizing due to an acute increase in ROS concentration. The oxidative stress hinders and disturbs the cellular metabolism and regulatory pathways, including the replication of DNA, transcription of RNA, and translation of proteins [13]. The response by a cell to counter oxidative stress to survive is the CDR, and it is present in the four domains of life.
Bacteria
The CDR of aerobic bacteria to combat oxidative stress is regulated by two players, a sensor for superoxide anion (SoxRS) and a sensor for hydrogen peroxide (OxyR) [8]. The antioxidant systems comprising SoxRS and OxyR lead the CDR for bacteria. Oxidative stress caused by superoxide anion is tackled by SoxRS, whereas OxyR responds to the oxidative stress induced by hydrogen peroxide [14].
Fungi
Most fungi are obligate aerobes and use aerobic metabolism. Yeast are well-studied fungi for the oxidative stress model. Yeast activation protein 1 (Yap1p), a transcription factor, leads the CDR in yeast against oxidative stress [8]. Yap1p comes from the family of activation proteins (AP1), which are evolutionarily conserved, and in mammalian cells, AP1 proteins are known to be players of CDR against different kinds of stresses [15]. In yeast, the binding of Yap1p in promoter regions with specific DNA sequences activates the transcription of genes known to respond to oxidative stress, reflecting the crucial role of mitochondria in the rising tide of chronic illness [16].
Plants
Plants are unique, as they cannot move when exposed to environmental damage. The by-product of aerobic metabolism in plants is ROS. ROS imbalance causes oxidative stress [17]. Studies have shown that the salicylic acid (SA)-induced response guides plants' CDR against oxidative stress [18]. Additionally, the SA system also regulates plant immunity [18].
Animals
One of the most significant causes of aging is excessive oxidative stress. The CDR may also play a significant role [3]. The more an animal produces ROS, the earlier it ages and dies, a phenomenon closely linked to the metabolic features of the cell danger response. Naked mole rats (NMRs) make a similar amount of ROS as mice, but their average lifespan is ten times more than that of mice (a mouse's average lifespan is three years, while NMR is 30 years) [8]. The key to NMRs being an outlier might be their efficient CDR, which mitigates and copes with oxidative stress [19]. NMRs are not the only exceptions to overcome the oxidative stress aging theory; other animals include birds and bats [20,21].
Humans
A study compared how the human cell responds to multiple stress situations [6]. The authors exposed the cells to five different types of stresses: oxidative stress, osmotic stress, heat stress (42 °C), ultraviolet stress, and proteasomal inhibition. They observed that the CDR responds to the stresses in specific patterns of ubiquitination to a different form of stress. One of the critical responses to heat stress is the broad shutdown of the translation process. The study observed that the CDR of ubiquitination, a key process in cellular healing cycles, is crucial in reinitiating the translation process after the stress leading to the recovery of cells from heat shock-induced stress [6]. Another observation of the study was that the heat shock-induced stress ubiquitination response had a considerable effect on cholesterol metabolism [6].
Cell Danger Response Mechanisms in Humans
Scientific literature shows that cellular metabolism and other stress responses regulate the CDR. As seen in the above scientific examples, when basic metabolic features of the cell, including ATP synthesis, nucleotide metabolism, and other purinergic signaling, are perturbed, a stress signal is sent across the cell, which triggers an organized set of cellular responses to defend the cell.
Metabolism integrates the combination of triggers (chemical, physical, or microbial threats) and regulates the CDR. The brain coordinates the CDR via metabolism and chemosensory integration of the whole body. Studies have shown that chronic illness may result from the abnormal persistence of the CDR [1,22].
Mitochondria are commonly known as “the powerhouse of the cell,” given their central role in cellular metabolism and energy production [23]. Mitochondria mainly observe and respond to the changes in the cellular environment (like the canary, see below!). Thus, mitochondria act as a fundamental regulator of the CDR by sensing cell safety and danger [1]. The prime movers of the CDR are small molecules, nutrients, and metabolites.
Mitochondria and the Cell Danger Response – the “Canary in the Coal Mine” for Cellular Stress
In the early to late 20th century, coal miners used the canary bird to detect carbon monoxide in the mines. The canary is highly sensitive to changes in oxygen in the environment, and an increase in carbon monoxide would cause their death. The canary acted as the “danger alarm system for coal miners.”
Similarly, studies show that mitochondria, the chief regulators of CDR, act as a “danger alarm system” for cellular stress [1,24,25]. A study from the Salk Institute found that mitochondria set off a molecular alarm when exposed to a stress or chemical that could potentially damage DNA [26]. The authors of the study investigated the response of mitochondria to the chemotherapeutic agent doxorubicin. They found that the stress caused by chemotherapeutic agents causes the release of mitochondrial DNA (mtDNA) in the cytoplasm. The release of mtDNA elicits the innate immune response that enhances nuclear DNA repair in cells and tissues. Thus, making the cell more chemoresistant [26]. Dr. Gerald Shadel, the corresponding author of the study, had this to say, “Mitochondria are acting as a first line of defense in sensing DNA stress. The mitochondria tell the rest of the cell, ‘Hey, I’m under attack, you better protect yourself.' [27]"
The Cell Danger Response In Action
In the landmark paper on CDR, famous scientist Robert Naviaux observed that when the cell is under stress and the CDR is active, the CDR will push the cells to take the following actions for survival [1]:
- It shifts cells from anabolism to catabolism.
- It changes the cell membrane fluidity to limit the damaged area of the cell.
- It releases antiviral and antimicrobial chemicals into the pericellular environment.
- It increases autophagy and mitochondrial fission to remove intracellular pathogens.
- It alters the epigenetics to change gene expression.
- It mobilizes endogenous retroviruses and other mobile genetic elements like the long interspersed nuclear elements (LINEs) to produce genetic variations.
- It sends signals to the neighboring and distant cells about the danger.
- It alters the host's behavior to prevent infection spread to kin and sleep patterns to facilitate healing.
The Rising Tide of Chronic Disease Associated with Persistent Cell Danger Response Activation
Recent scientific developments have provided proof of persistent CDR with many chronic diseases [24,25,28-31]. Mitochondria trigger the persistent CDR that reaches the brain. With its feedback loop using the autocrine and neuroendocrine system, the brain amplifies the CDR to eradicate the threat and ensure safety. If the cells fail to remove the threat, an activated CDR will persist, cause a form of anxiety in the cellular environment, and could become a source of chronic disease [25].
Chronic Fatigue Syndrome
Chronic fatigue syndrome (CFS) is a disease of extreme fatigue with a negative impact on cognition and multiple organ systems [32]. The mechanism of this disease is not well understood and is mainly considered a psychological illness. A study by Dr. Robert Naviaux recently showed that CFS is indeed a metabolic disorder and results from persistent CDR [30]. In his research, he observed that constituents of the CDR pathway represented 80% of the abnormal metabolites of CFS, proving CFS to be a metabolic disorder.
Autism
Autism is a neurodegenerative disorder. Emerging evidence suggests a strong link between mitochondrial dysfunction and autism spectrum disorder, pointing towards a new science that connects environmental health and chronic disease pathogenesis and treatment [33]. Studies have observed that abnormal ATP signaling causes mitochondrial dysfunction [33]. In a small clinical trial, ten children who have autism were treated with a dose of a drug to inhibit ATP [34]. The study found a significant change in the communication and social behavior of the child when the abnormal ATP signaling was silenced.
Many chronic diseases, including melanoma, post-traumatic stress disorder, and others, are associated with the CDR, highlighting the developmental and autoimmune potential when cell danger response is not properly regulated. An exhaustive list and detailed mechanisms of their association with the CDR can be accessed in this 2019 review [31].
How to Improve Mitochondrial Function to Improve the Cell Danger Response
The CDR is regulated and controlled by mitochondria. Hence, it is vital that we keep our mitochondria healthy. Dr. Joseph Pizzorno, scientist and author of the best-seller “The Toxin Solution,” suggests five strategies to improve mitochondrial function [35]:
- Optimize nutrient status to limit oxygen and high-energy electron leakage in the electron transport chain
- Decrease toxin exposure
- Provide nutrients that protect the mitochondria from oxidative stress
- Utilize nutrients that facilitate mitochondrial ATP production
- Build muscle mass
And a bonus!
- Just Blue
Just Blue is 16 mg of pure pharmaceutical grade methylene blue, a compound well-known as an electron cycler, donating electrons to the electron transport chain and then scavenging the mitochondria and cytosol for free radicals/ROS.
The end result? Enhanced ATP production and enhanced antioxidant protection, both of which are essential in the activation and regulation of the cell danger response.
Give your mitochondria some Just Blue lovin’ so your CDR is at the ready!
But here are a few caveats:
Methylene blue concentrates in the urine and will turn urine blue (a benign side effect). It is also not recommended in pregnant and breastfeeding women, those with G6PD deficiency, or those taking SSRI or SNRI medications (the latter unless under close practitioner supervision). Also, watch out for combining it with high-dose psychedelics due to their action on serotonin receptors.
References
- Naviaux, R.K. Metabolic Features of the Cell Danger Response. Mitochondrion 2014, 16, 7–17, doi:10.1016/j.mito.2013.08.006.
- Blaustein, R. The Great Oxidation Event. Bioscience 2016, 66, 189–195, doi:10.1093/biosci/biv193.
- Valera-Alberni, M.; Canto, C. Mitochondrial Stress Management: A Dynamic Journey. Cell Stress 2018, 2, 253–274, doi:10.15698/cst2018.10.158.
- Tytell, M.; Hooper, P.L. Heat Shock Proteins: New Keys to the Development of Cytoprotective Therapies. Expert Opin. Ther. Targets 2001, 5, 267–287, doi:10.1517/14728222.5.2.267.
- Zhou, R.; Yazdi, A.S.; Menu, P.; Tschopp, J. A Role for Mitochondria in NLRP3 Inflammasome Activation. Nature 2011, 469, 221–226, doi:10.1038/nature09663.
- Maxwell, B.A.; Gwon, Y.; Mishra, A.; Peng, J.; Nakamura, H.; Zhang, K.; Kim, H.J.; Taylor, J.P. Ubiquitination Is Essential for Recovery of Cellular Activities after Heat Shock. Science (80-. ). 2021, 372, doi:10.1126/science.abc3593.
- West, A.P.; Shadel, G.S.; Ghosh, S. Mitochondria in Innate Immune Responses. Nat. Rev. Immunol. 2011, 11, 389–402, doi:10.1038/nri2975.
- Lushchak, V.I. Adaptive Response to Oxidative Stress: Bacteria, Fungi, Plants and Animals. Comp. Biochem. Physiol. - C Toxicol. Pharmacol. 2011, 153, 175–190, doi:10.1016/j.cbpc.2010.10.004.
- Naviaux, R.K. Oxidative Shielding or Oxidative Stress? J. Pharmacol. Exp. Ther. 2012, 342, 608–618, doi:10.1124/jpet.112.192120.
- Haynes, C.M.; Fiorese, C.J.; Lin, Y.F. Evaluating and Responding to Mitochondrial Dysfunction: The Mitochondrial Unfolded-Protein Response and Beyond. Trends Cell Biol. 2013, 23, 311–318, doi:10.1016/j.tcb.2013.02.002.
- Lee, A.H.; Glimcher, L.H. Intersection of the Unfolded Protein Response and Hepatic Lipid Metabolism. Cell. Mol. Life Sci. 2009, 66, 2835–2850.
- Silva, J.M.; Wong, A.; Carelli, V.; Cortopassi, G.A. Inhibition of Mitochondrial Function Induces an Integrated Stress Response in Oligodendroglia. Neurobiol. Dis. 2009, 34, 357–365, doi:10.1016/j.nbd.2009.02.005.
- Oktyabrsky, O.N.; Smirnova, G. V. Redox Regulation of Cellular Functions. Biochem. 2007, 72, 132–145, doi:10.1134/S0006297907020022.
- Seo, S.W.; Kim, D.; Szubin, R.; Palsson, B.O. Genome-Wide Reconstruction of OxyR and SoxRS Transcriptional Regulatory Networks under Oxidative Stress in Escherichia Coli K-12 MG1655. Cell Rep. 2015, 12, 1289–1299, doi:10.1016/j.celrep.2015.07.043.
- Lushchak, V.I. Oxidative Stress in Yeast. Biochem. 2010, 75, 281–296, doi:10.1134/S0006297910030041.
- Toone, W.M.; Jones, N. AP-1 Transcription Factors in Yeast. Curr. Opin. Genet. Dev. 1999, 9, 55–61, doi:10.1016/S0959-437X(99)80008-2.
- Demidchik, V. Mechanisms of Oxidative Stress in Plants: From Classical Chemistry to Cell Biology. Environ. Exp. Bot. 2015, 109, 212–228, doi:10.1016/j.envexpbot.2014.06.021.
- Saleem, M.; Fariduddin, Q.; Castroverde, C.D.M. Salicylic Acid: A Key Regulator of Redox Signalling and Plant Immunity. Plant Physiol. Biochem. 2021, 168, 381–397, doi:10.1016/j.plaphy.2021.10.011.
- Saldmann, F.; Viltard, M.; Leroy, C.; Friedlander, G. The Naked Mole Rat: A Unique Example of Positive Oxidative Stress. Oxid. Med. Cell. Longev. 2019, 2019, doi:10.1155/2019/4502819.
- Costantini, D. Oxidative Stress in Ecology and Evolution: Lessons from Avian Studies. Ecol. Lett. 2008, 11, 1238–1251, doi:10.1111/j.1461-0248.2008.01246.x.
- Selman, C.; Blount, J.D.; Nussey, D.H.; Speakman, J.R. Oxidative Damage, Ageing, and Life-History Evolution: Where Now? Trends Ecol. Evol. 2012, 27, 570–577, doi:10.1016/j.tree.2012.06.006.
- Naviaux, J.C.; Wang, L.; Li, K.; Bright, A.T.; Alaynick, W.A.; Williams, K.R.; Powell, S.B.; Naviaux, R.K. Antipurinergic Therapy Corrects the Autism-like Features in the Fragile X (Fmr1 Knockout) Mouse Model. Mol. Autism 2015, 6, doi:10.1186/2040-2392-6-1.
- Ma, C.; Xia, F.; Kelley, S.O. Mitochondrial Targeting of Probes and Therapeutics to the Powerhouse of the Cell. Bioconjug. Chem. 2020, 31, 2650–2667, doi:10.1021/acs.bioconjchem.0c00470.
- Naviaux, R.K. Incomplete Healing as a Cause of Aging: The Role of Mitochondria and the Cell Danger Response. Biology (Basel). 2019, 8, doi:10.3390/biology8020027.
- Naviaux, R.K. Perspective: Cell Danger Response Biology—The New Science That Connects Environmental Health with Mitochondria and the Rising Tide of Chronic Illness. Mitochondrion 2020, 51, 40–45, doi:10.1016/j.mito.2019.12.005.
- Wu, Z.; Oeck, S.; West, A.P.; Mangalhara, K.C.; Sainz, A.G.; Newman, L.E.; Zhang, X.O.; Wu, L.; Yan, Q.; Bosenberg, M.; et al. Mitochondrial DNA Stress Signalling Protects the Nuclear Genome. Nat. Metab. 2019, 1, 1209–1218, doi:10.1038/s42255-019-0150-8.
- News, S. Mitochondria Are the “Canary in the Coal Mine” for Cellular Stress Available online: https://www.salk.edu/news-release/mitochondria-are-the-canary-in-the-coal-mine-for-cellular-stress/.
- Naviaux, R.K.; Zolkipli, Z.; Wang, L.; Nakayama, T.; Naviaux, J.C.; Le, T.P.; Schuchbauer, M.A.; Rogac, M.; Tang, Q.; Dugan, L.L.; et al. Antipurinergic Therapy Corrects the Autism-Like Features in the Poly(IC) Mouse Model. PLoS One 2013, 8, doi:10.1371/journal.pone.0057380.
- Naviaux, J.C.; Schuchbauer, M.A.; Li, K.; Wang, L.; Risbrough, V.B.; Powell, S.B.; Naviaux, R.K. Reversal of Autism-like Behaviors and Metabolism in Adult Mice with Single-Dose Antipurinergic Therapy. Transl. Psychiatry 2014, 4, doi:10.1038/tp.2014.33.
- Naviaux, R.K.; Naviaux, J.C.; Li, K.; Bright, A.T.; Alaynick, W.A.; Wang, L.; Baxter, A.; Nathan, N.; Anderson, W.; Gordon, E. Metabolic Features of Chronic Fatigue Syndrome. Proc. Natl. Acad. Sci. U. S. A. 2016, 113, E5472–E5480, doi:10.1073/pnas.1607571113.
- Naviaux, R.K. Metabolic Features and Regulation of the Healing Cycle—A New Model for Chronic Disease Pathogenesis and Treatment. Mitochondrion 2019, 46, 278–297, doi:10.1016/j.mito.2018.08.001.
- Close, S.; Marshall-Gradisnik, S.; Byrnes, J.; Smith, P.; Nghiem, S.; Staines, D. The Economic Impacts of Myalgic Encephalomyelitis/Chronic Fatigue Syndrome in an Australian Cohort. Front. Public Heal. 2020, 8, 1–8, doi:10.3389/fpubh.2020.00420.
- Siddiqui, M.F.; Elwell, C.; Johnson, M.H. ECSA 141210_e_bulletin.Pdf. 2016, 6, doi:10.4172/2165-7890.1000190.Mitochondrial.
- Naviaux, R.K.; Curtis, B.; Li, K.; Naviaux, J.C.; Bright, A.T.; Reiner, G.E.; Westerfield, M.; Goh, S.; Alaynick, W.A.; Wang, L.; et al. Low-Dose Suramin in Autism Spectrum Disorder: A Small, Phase I/II, Randomized Clinical Trial. Ann. Clin. Transl. Neurol. 2017, 4, 491–505, doi:10.1002/acn3.424.
- Pizzorno, J. Mitochondria—Fundamental to Life and Health THE PATH AHEAD. Integr. Med. A Clin. J. 2014, 13, 8–15.
Comments (0)
There are no comments for this article. Be the first one to leave a message!