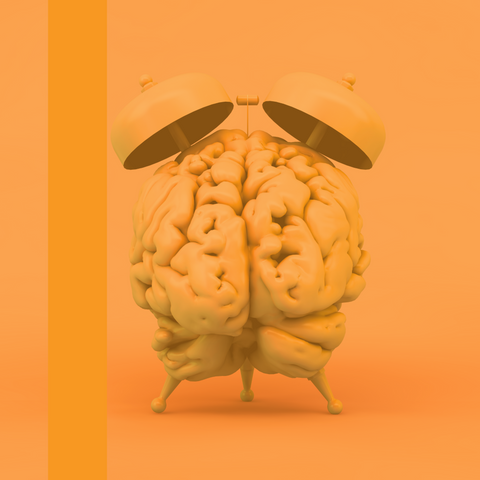
You may already be familiar with the glymphatic system if you read our previous blog article, but in case you haven’t, don’t worry! We’ll give you a quick recap of its discovery and how it works in this article.
We’ll also be discussing the glymphatic system’s connections to sleep, arterial pulsation, respiration, posture, and aging, external factors that decrease its clearance activity and how we can remedy or prevent them, and its implications in some neurological disorders.
Discovery of the glymphatic system
The lymphatic system plays a key role in the body's immune defense and fluid balance maintenance. It correlates with the rate of tissue metabolism and enables the clearance of interstitial fluid (ISF) with its constituent proteins and other solutes not absorbed across post-capillary small veins. Although the central nervous system (CNS), composed of the spinal cord and brain, is characterized by very high metabolic rates, it lacks the lymphatic system. The cerebrospinal fluid (CSF) has long been thought to play a role in solute clearance in the brain. However, the brain-clearance system remained unknown until a decade ago.
In 2012, Iliff and colleagues observed a flow of subarachnoid CSF into and through the brain interstitium using two-photon imaging in the brains of live mice for the first time. This CSF influx into the parenchyma then flushed out waste from the brain with ISF efflux through perivascular channels formed by astroglial cells. They demonstrated that the aquaporin-4 receptors (AQP4), providing fast water transportation in the CNS, support CSF influx and facilitate ISF efflux with bulk solute clearance [1].
Due to its dependence on astroglial cells and its similarity to the peripheral lymphatic system, Iliff and colleagues named that system the “glial-lymphatic” or glymphatic system. Since then, many studies have observed the glymphatic system in the human brain [2,3].
The brain-clearance system
Neurons are susceptible to their environment and rapidly produce large amounts of waste products as a result of their normal metabolism that could trigger irreversible neuronal injury and, ultimately, prevent synaptic transmission [4]. Thus, fast and efficient removal of waste products is essential in the brain. The main function of the glymphatic system is to remove between 40 and 80% of soluble proteins and metabolites (including neurotoxic compounds such as β-amyloid, tau, and α‐synuclein) from the brain interstitial space via its unique paravascular tunnel system to ensure the proper functioning of neurons [5]. It then connects with the peripheral lymphatic system and enables the CNS-soluble waste to be processed and degraded by the liver [6]. In addition, the glymphatic system helps deliver essential molecules for normal brain activity such as nutrients (glucose, amino acids, and lipids), growth factors, and neuromodulators [4,5,7,8].
The glymphatic system’s connections to sleep and more
The glymphatic system mainly functions during sleep and seems largely disengaged during wakefulness. Research suggests that sleep helps optimize the system, enabling more effective waste clearance. Indeed, the interstitial space increases by more than 60% during sleep, resulting in a striking increase in the exchange of CSF with ISF and leading to the efficient clearance of β-amyloid as well as other compounds from the brain [9].
As a result, sleep is considered the most fundamental element of the glymphatic system activity, particularly slow-wave sleep during non-rapid eye movement (NREM) sleep [10]. Both sleep duration and quality influence the glymphatic system as sleep disruption results in glymphatic clearance impairment and compromises the essential removal of extracellular metabolites from the brain [9]. Indeed, after acute sleep deprivation or slow-wave sleep disruption, studies report an increase in β-amyloid levels in the brain [11]. Sleep deprivation has also been shown to alter the expression of AQP4 [12]. Self-reported sleep duration was demonstrated to correlate with β-amyloid accumulation [13] and, finally, poor sleep quality over several days was shown to increase tau in the brain [14]. These findings highlight the importance of adequate and quality sleep for overall brain health.
Besides, arterial pulsation, respiration, and posture may also regulate the glymphatic system. Cardiovascular pulsation can be observed in CSF flow in periarterial spaces of the brain [15], and dobutamine, a β‐adrenergic agonist, was shown, apart from increasing pulsatility of arteries, to stimulate CSF flow as well as glymphatic influx [16]. On the other hand, the glymphatic ISF efflux is strongly dependent on intraventricular CSF flow, which, in turn, depends on respiration [17] and posture during sleep [10,18].
Finally, aging greatly reduces the glymphatic system activity. A study on mice showed a dramatic reduction of brain clearance in aged compared to young mice, along with a significant reduction of arterial pulsation in the vessel walls, loss of AQP4 polarization (or loss of the specific AQP4 distribution to the perivascular endfeet of astroglial cells), and decline in CSF-ISF exchange [19]. This age‐dependent decrease in glymphatic system clearance was also observed in humans when comparing older patients to younger individuals using MRI tracers [20].
External factors that decrease the glymphatic system's clearance activity
The glymphatic activity can also be affected by external factors. For example, inhaled isoflurane, an anesthetic used to start or maintain general anesthesia, had a negative impact on the intracranial CSF circulation, and consequently disrupted glymphatic activity at high doses in mice [21,22]. Another study in mice showed that cocaine treatment greatly hindered glymphatic activity, as evidenced by reduced CSF influx and decreased ISF efflux of interstitial molecules out of the parenchyma [23]. In humans, benzodiazepines, a drug family used to treat anxiety, insomnia, and seizures, are well-known for reducing slow-wave activity during sleep. As a result, they are suspected to interfere with glymphatic activity [24].
Due to its recent discovery, key structural and functional aspects — such as the functional differences between awake and sleep states, and the nature (active or passive) of the CSF flow — are still not fully understood and require further investigation. Despite these uncertainties, the glymphatic system is widely acknowledged for playing a significant role in a variety of neurological disorders [4].
The glymphatic system and neurological disorders
Alzheimer’s disease
Alzheimer’s disease (AD) is the most common type of dementia in individuals aged 65 years or older. It is characterized by the presence of amyloid plaques (composed of β-amyloid) and neurofibrillary tangles (containing hyperphosphorylated tau) in the brains of patients [10].
Although its early pathogenesis is unknown, sleep disturbances seem to be a common element in the varied clinical, imaging, and laboratory manifestations of AD. These sleep disturbances are implicated in hindering the glymphatic system flow in patients with AD and dementia [25,26], which participates in β-amyloid and tau protein accumulation that may, ultimately, be a factor favoring their aggregation. AQP4 expression and polarization were also altered in individuals with AD when compared with cognitively intact elderly individuals [27]. Consequently, increasing glymphatic activity by modifying sleep patterns and improving sleep quality may hold promise in slowing AD progression [10].
Traumatic brain injury
After a traumatic brain injury (TBI), sleeping problems are very common and 68% of patients develop sleeping impairments or abnormal sleep patterns. Neurofibrillary tangles of tau aggregates typically observed in AD are also frequently present in TBI.
Researchers observed that TBI caused AQP4 mislocalization in mice, which could significantly disrupt the glymphatic system [28]. They also measured the glymphatic activity following TBI and found that its clearance function was reduced by 60% for at least one month after the injury, contributing to the accumulation of tau in the brain [29].
Other studies showed that the glymphatic system plays a central role in the pathophysiological process of stroke, a subtype of TBI, encompassing brain edema, blood-brain barrier disruption, neuroinflammation, and neuronal death [30,31].
Epilepsy
Epilepsy is a common condition affecting approximately 50 million people worldwide. It is characterized by seizures, resulting from uncontrolled electrical activity between brain cells and causing temporary changes in behavior, movements, and feelings. Sleep disturbances are also common among epilepsy patients and increase the risk of seizures [32,33]. The glymphatic system is likely to be involved in epilepsy as several studies reported β-amyloid, tau, and α‐synuclein depositions in temporal lobe epilepsy patients suggesting cerebral waste accumulation and glymphatic system impairment [17,34-36].
Parkinson’s disease
Parkinson’s disease (PD) is a progressive neurodegenerative condition impacting approximately 9.4 million people worldwide. It presents with rigidity and tremors, leading to diminished movement, slow speech, articulation difficulties, sleep disturbances, and cognitive decline. PD is characterized by the loss of dopaminergic neurons in the substantia nigra of the brain and the presence of α-synuclein aggregates, called Lewy bodies, in the surviving neurons [37].
Researchers demonstrated a close interaction between AQP4 and parenchymal α-synuclein in mice. They found that AQP4 deficiency accelerated α-synuclein accumulation, facilitated the loss of dopaminergic neurons, and accelerated the apparition of PD-like symptoms. On the other hand, they observed that overexpression of α-synuclein reduced the expression/polarization of AQP4 and suppressed glymphatic activity [38].
Cognitive impairment in PD is linked to significant β-amyloid, tau, and α-synuclein deposition in the brain parenchyma [39-41]. Autopsy and cytology studies revealed that β-amyloid, tau, and α-synuclein could mutually promote deposition [42,43]. As a result, improving glymphatic activity may decelerate cognitive impairment in PD and represent a new perspective for future research [37].
Conclusion
The glymphatic system has been at the center of intense research since its discovery in 2012. Given its implications in neurological disorders, it opens new perspectives to treat or slow the progression of many disorders. As its brain-clearance activity is very closely related to sleep, the glymphatic system could explain the vital importance of sleep — a phenomenon that has intrigued researchers for many decades.
In conclusion, it's never too late to prioritize your sleep, and here at Troscriptions, we can help you with that! Tro Zzz is our complete sleep solution troche that helps you fall asleep, stay asleep, and wake up feeling refreshed. Try it now and refresh your glymphatic system!
References
- Iliff, J. J. & Wang, M. & Liao, Y. et al. (2012) A Paravascular Pathway Facilitates CSF Flow Through the Brain Parenchyma and the Clearance of Interstitial Solutes, Including Amyloid β. Science Translational Medicine, 4(147), 147-111. http://dx.doi.org/10.1126/scitranslmed.3003748
- Eide, P. K. & Ringstad, G. (2015) MRI with intrathecal MRI gadolinium contrast medium administration: a possible method to assess glymphatic function in the human brain. Acta Radiologica Open, 4(11), 205846011560963. http://dx.doi.org/10.1177/2058460115609635
- Ringstad, G. & Vatnehol, S. A. S. & Eide, P. K. (2017) Glymphatic MRI in idiopathic normal pressure hydrocephalus. Brain, 140(10), 2691-2705. http://dx.doi.org/10.1093/brain/awx191
- Christensen, J. & Yamakawa, G. R. & Shultz, S. R. et al. (2021) Is the glymphatic system the missing link between sleep impairments and neurological disorders? Examining the implications and uncertainties. Progress in Neurobiology, 198101917. http://dx.doi.org/10.1016/j.pneurobio.2020.101917
- Jessen, N. A. & Munk, A. S. F. & Lundgaard, I. et al. (2015) The Glymphatic System: A Beginner’s Guide. Neurochemical Research, 40(12), 2583-2599. http://dx.doi.org/10.1007/s11064-015-1581-6
- Aspelund, A. & Antila, S. & Proulx, S. T. et al. (2015) A dural lymphatic vascular system that drains brain interstitial fluid and macromolecules. Journal of Experimental Medicine, 212(7), 991-999. http://dx.doi.org/10.1084/jem.20142290
- Lundgaard, I. & Li, B. & Xie, L. et al. (2015) Direct neuronal glucose uptake heralds activity-dependent increases in cerebral metabolism. Nature Communications, 6(1), 6807. http://dx.doi.org/10.1038/ncomms7807
- Thrane, V. R. & Thrane, A. S. & Plog, B. A. et al. (2013) Paravascular microcirculation facilitates rapid lipid transport and astrocyte signaling in the brain. Scientific Reports, 3(1),
- http://dx.doi.org/10.1038/srep02582
- Xie, L. & Kang, H. & Xu, Q. et al. (2013) Sleep Drives Metabolite Clearance from the Adult Brain. Science, 342(6156), 373-377. http://dx.doi.org/10.1126/science.1241224
- Astara, K. & Tsimpolis, A. & Kalafatakis, K. et al. (2024) Sleep disorders and Alzheimer’s disease pathophysiology: The role of the Glymphatic System. A scoping review. Mechanisms of Ageing and Development, 217111899. http://dx.doi.org/10.1016/j.mad.2023.111899
- Ooms, S. & Overeem, S. & Besse, K. et al. (2014) Effect of 1 Night of Total Sleep Deprivation on Cerebrospinal Fluid β-Amyloid 42 in Healthy Middle-Aged Men: A Randomized Clinical Trial. JAMA Neurology, 71(8), 971. http://dx.doi.org/10.1001/jamaneurol.2014.1173
- Wang, Y. & Huang, C. & Guo, Q. et al. (2022) Aquaporin-4 and Cognitive Disorders. Aging and disease, 13(1), 61. http://dx.doi.org/10.14336/ad.2021.0731
- Spira, A. P. & Gamaldo, A. A. & An, Y. et al. (2013) Self-reported Sleep and β-Amyloid Deposition in Community-Dwelling Older Adults. JAMA Neurology, 70(12), 1537-43. http://dx.doi.org/10.1001/jamaneurol.2013.4258
- Ju, Y.-E. S. & Ooms, S. J. & Sutphen, C. et al. (2017) Slow wave sleep disruption increases cerebrospinal fluid amyloid-β levels. Brain, 140(8), 2104-2111. http://dx.doi.org/10.1093/brain/awx148
- Kiviniemi, V. & Wang, X. & Korhonen, V. et al. (2015) Ultra-fast magnetic resonance encephalography of physiological brain activity – Glymphatic pulsation mechanisms?. Journal of Cerebral Blood Flow & Metabolism, 36(6), 1033-1045. http://dx.doi.org/10.1177/0271678x15622047
- Iliff, J. J. & Wang, M. & Zeppenfeld, D. M. et al. (2013) Cerebral Arterial Pulsation Drives Paravascular CSF–Interstitial Fluid Exchange in the Murine Brain. The Journal of Neuroscience, 33(46), 18190-18199. http://dx.doi.org/10.1523/jneurosci.1592-13.2013
- Nowicka, D. (2023) Glymphatic system and aquaporin4 in epilepsy. Acta Neurobiologiae Experimentalis, 83(4), 117-128. http://dx.doi.org/10.55782/ane-2023-2498
- Lee, H. & Xie, L. & Yu, M. et al. (2015) The Effect of Body Posture on Brain Glymphatic Transport. Journal of Neuroscience, 35(31), 11034-11044. http://dx.doi.org/10.1523/jneurosci.1625-15.2015
- Kress, B. T. & Iliff, J. J. & Xia, M. et al. (2014) Impairment of paravascular clearance pathways in the aging brain. Annals of Neurology, 76(6), 845-861. http://dx.doi.org/10.1002/ana.24271
- Zhou, Y. & Cai, J. & Zhang, W. et al. (2020) Impairment of the Glymphatic Pathway and Putative Meningeal Lymphatic Vessels in the Aging Human. Annals of Neurology, 87(3), 357-369. http://dx.doi.org/10.1002/ana.25670
- Gakuba, C. & Gaberel, T. & Goursaud, S. et al. (2018) General Anesthesia Inhibits the Activity of the “Glymphatic System”. Theranostics, 8(3), 710-722. http://dx.doi.org/10.7150/thno.19154
- Ren, X. & Liu, S. & Lian, C. et al. (2021) Dysfunction of the Glymphatic System as a Potential Mechanism of Perioperative Neurocognitive Disorders. Frontiers in Aging Neuroscience, 13659457. http://dx.doi.org/10.3389/fnagi.2021.659457
- Chen, W. & Huang, P. & Zeng, H. et al. (2020) Cocaine-induced structural and functional impairments of the glymphatic pathway in mice. Brain, Behavior, and Immunity, 8897-104. http://dx.doi.org/10.1016/j.bbi.2020.04.057
- Lancel, M. (1999) Role of GABAA Receptors in the Regulation of Sleep: Initial Sleep Responses to Peripherally Administered Modulators and Agonists. Sleep, 22(1), 33-42. http://dx.doi.org/10.1093/sleep/22.1.33
- Taoka, T. & Masutani, Y. & Kawai, H. et al. (2017) Evaluation of glymphatic system activity with the diffusion MR technique: diffusion tensor image analysis along the perivascular space (DTI-ALPS) in Alzheimer’s disease cases. Japanese Journal of Radiology, 35(4), 172-178. http://dx.doi.org/10.1007/s11604-017-0617-z
- Ringstad, G. & Valnes, L. M. & Dale, A. M. et al. (2018) Brain-wide glymphatic enhancement and clearance in humans assessed with MRI. JCI Insight, 3(13), e121537. http://dx.doi.org/10.1172/jci.insight.121537
- Zeppenfeld, D. M. & Simon, M. & Haswell, J. D. et al. (2017) Association of Perivascular Localization of Aquaporin-4 With Cognition and Alzheimer's Disease in Aging Brains. JAMA Neurology, 74(1), 91-99. http://dx.doi.org/10.1001/jamaneurol.2016.4370
- Gomolka, R. S. & Hablitz, L. M. & Mestre, H. et al. (2023) Loss of aquaporin-4 results in glymphatic system dysfunction via brain-wide interstitial fluid stagnation. eLife, 12e82232. http://dx.doi.org/10.7554/elife.82232
- Iliff, J. J. & Chen, M. J. & Plog, B. A. et al. (2014) Impairment of Glymphatic Pathway Function Promotes Tau Pathology after Traumatic Brain Injury. The Journal of Neuroscience, 34(49), 16180-16193. http://dx.doi.org/10.1523/jneurosci.3020-14.2014
- Lv, T. & Zhao, B. & Hu, Q. et al. (2021) The Glymphatic System: A Novel Therapeutic Target for Stroke Treatment. Frontiers in Aging Neuroscience, 13689098. http://dx.doi.org/10.3389/fnagi.2021.689098
- Ji, C. & Yu, X. & Xu, W. et al. (2021) The role of glymphatic system in the cerebral edema formation after ischemic stroke. Experimental Neurology, 340113685. http://dx.doi.org/10.1016/j.expneurol.2021.113685
- Bazil, C. W. (2003) Epilepsy and sleep disturbance. Epilepsy Behavior, 439-45. http://dx.doi.org/10.1016/j.yebeh.2003.07.005
- Dell’Aquila, J. T. & Soti, V. (2022) Sleep deprivation: a risk for epileptic seizures. Sleep Science, 15(02), 245-249. http://dx.doi.org/10.5935/1984-0063.20220046
- Yang, J. W. & Czech, T. & Felizardo, M. et al. (2006) Aberrant expression of cytoskeleton proteins in hippocampus from patients with mesial temporal lobe epilepsy. Amino Acids, 30(4), 477-493. http://dx.doi.org/10.1007/s00726-005-0281-y
- Tai, X. Y. & Koepp, M. & Duncan, J. S. et al. (2016) Hyperphosphorylated tau in patients with refractory epilepsy correlate with cognitive decline: a study of temporal lobe resections. Brain, 139(9), 2441-2455. http://dx.doi.org/10.1093/brain/aww187
- Paudel, Y. N. & Angelopoulou, E. & Piperi, C. et al. (2020) Revisiting the Impact of Neurodegenerative Proteins in Epilepsy: Focus on Alpha-Synuclein, Beta-Amyloid, and Tau. Biology, 9(6), 122. http://dx.doi.org/10.3390/biology9060122
- Si, X. & Guo, T. & Wang, Z. et al. (2022) Neuroimaging evidence of glymphatic system dysfunction in possible REM sleep behavior disorder and Parkinson’s disease. NPJ Parkinson’s Disease, 8(1), 54. http://dx.doi.org/10.1038/s41531-022-00316-9
- Zhang, Y. & Zhang, C. & He, X.-Z. et al. (2023) Interaction Between the Glymphatic System and α-Synuclein in Parkinson’s Disease. Molecular Neurobiology, 60(4), 2209-
- http://dx.doi.org/10.1007/s12035-023-03212-2
- Halliday, G. M. & Leverenz, J. B. & Schneider, J. S. et al. (2014) The neurobiological basis of cognitive impairment in Parkinson’s disease. Movement Disorders, 29(5), 634-
- http://dx.doi.org/10.1002/mds.25857
- Irwin, D. J. & Lee, V. M.-Y. & Trojanowski, J. Q. (2013) Parkinson’s disease dementia: convergence of α-synuclein, tau, and amyloid-β pathologies. Nature Reviews Neuroscience, 14(9), 626-636. http://dx.doi.org/10.1038/nrn3549
Comments (0)
There are no comments for this article. Be the first one to leave a message!