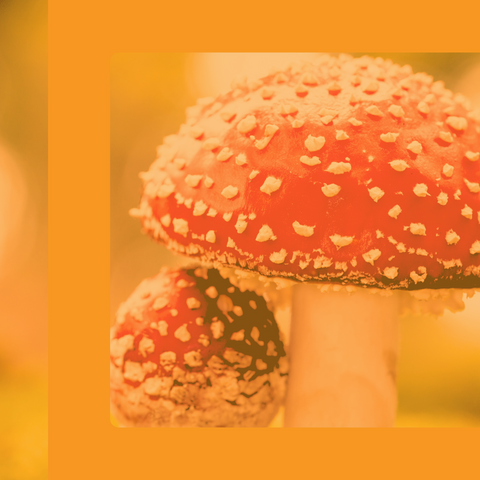
Imagine a beautiful mushroom. Do you see a dome-shaped cap atop a slender stem? Visualize the cap’s color. Is it, perhaps, a deep red adorned with white dots?
If so, you have envisioned the most commonly portrayed mushroom in mainstream culture Amanita muscaria, commonly known as the fly agaric.
Fairy tale forests abound with vibrant toadstools, occasionally serving as home to fantastical, magical creatures like the Smurfs — tiny, blue, gnome-like beings that live in a mushroom village. In Lewis Carroll’s “Alice in Wonderland,” a caterpillar puffing on a hookah encourages Alice to eat mushrooms, prompting her to grow in size. Mario, the most iconic video game character, embarks on a mission to rescue the Mushroom Kingdom, aided by power-up mushrooms that confer superpowers and extra lives. Mushrooms imbued with magical capabilities. But where are these ideas derived from?
This article introduces the Amanita muscaria mushroom, tracing its captivating history and its influence on popular culture, and explores the effects caused by its principal bioactive compounds: ibotenic acid and agarin (also known as muscimol).
The Siberian Shamans
For millennia, humans have been using mushrooms for two primary reasons: sustenance and recreational enjoyment. In the 19th century, after centuries of isolation from the rest of Russia, the first explorers made their way to eastern Siberia. There, they encountered a Stone Age culture with strong connections to nature and a profound affinity for reindeer, from which they not only obtained meat, milk, and hides but also a spiritual connection. Members of the community dedicated to medicine were called “shamans.”
Shamans ventured into birch forests to gather fly agaric and used it in their rituals and curative practices to achieve altered states of consciousness. A remarkable practice involved the ingestion of urine from reindeer under the effects of fly agaric to experience the effects of the mushroom. The cycle continued as shamanic initiates consumed the urine of the shamans, given that the compounds were still active. Even in modern times, communities dwelling in Siberia such as the Ostyak and Vogul tribes in western Siberia, and the Kamchadal, Koryak, and Chukchi tribes in eastern Siberia still incorporate fly agaric into their shamanic ceremonies [1,2].
A Psychedelic Christmas Tale
Western Christmas mythology might have been influenced by Amanita muscaria and the practices of Siberian shamans. Some claim that Santa Claus is a depiction of a Siberian shaman clad in distinctive red and white hues reminiscent of the fly agaric. The reindeer that accompany Santa on his mythical journey may share profound ties with Siberian communities, given that these animals were essential to their economy.
In mainstream culture, the image of Rudolph and his companions traversing the skies to deliver Santa to every home in the world might have been constructed as an allegory for the mind-altering voyages induced by the consumption of Amanita muscaria.
Interestingly, these “magic” fungi were often hung from tree branches to dry out before eating, akin to Christmas tree decorations. The concept of Santa as a shaman bearing either hallucinogenic mushrooms or gift (the German word for “poison”) remains controversial and lacks solid evidence, but does not seem like a far-fetched theory [3,4].
Biology of Amanita muscaria
The name “fly agaric” comes from its ability to repel flies and has been used for this purpose for centuries [5]. Taxonomically, mushrooms within the genus Amanita belong to the division Basidiomycota, class Agaricomycetes, order Agaricales, and family Amanitaceae. This genus encompasses roughly 1000 species distributed worldwide, from which approximately 100 species exhibit toxicity [6]. Notably, certain species produce hallucinogenic alkaloids [7].
Amanita muscaria, the focus of our attention in this article, is one of these toxic mushrooms with hallucinogenic properties. Its cap spans 90-145 mm in diameter and can be red, orange, or yellow, covered with its characteristic white scales.
The gills under the cap (responsible for spore spreading) and the stipe are also white. The stipe is 60-210 × 8-22 mm and has a skirt-like annulus around it [8,9]. Geographically, Amanita muscaria is distributed across the northern hemisphere and most commonly found in symbiosis with birch, pine, spruce, and eucalyptus trees [10].
Chemical Composition of Amanita muscaria
Mushrooms are cherished ingredients in various cuisines, such as Mediterranean or Japanese cooking. Mushrooms are rich in proteins, fat, and carbohydrates, making them an excellent addition to a healthy, balanced diet. Still, a mushroom’s composition might include mycotoxins, a varied group of molecules that lead to intoxication and other harmful effects [7].
Mycotoxins may have a benefit, however, as we can gain insights into their molecular mechanisms, which could pave the way for the development of novel drugs to treat human diseases.
Now, let’s dive into the chemical composition of Amanita muscaria and its bioactive compounds!
Muscarine
Muscarine is a selective cholinergic agonist that was isolated in 1869 in the laboratory of Oswald Schmiedeberg, a distinguished pharmacologist of his generation [2]. It is an alkaloid long believed to be the main active principle responsible for the hallucinogenic properties of the fly agaric. While it contributes to some of the symptoms associated with the consumption of Amanita muscaria, it is now understood that the hallucinogenic effects originate from ibotenic acid and agarin. Muscarine can cause bradycardia, low blood pressure, increased bronchial secretions, tearing, vomiting, diarrhea, bronchospasm, salivation, pupil contraction, and blurred vision[7].
Antioxidants
Amanita muscaria contains an array of various bioactive compounds with demonstrated antioxidant activity. Among them are peptides (such as glutathione and ergothioneine), phenolic compounds (including flavonoids, lignans, oxidized polyphenols, phenolic acids, stilbenes, and tannins), vitamins and derivatives (like ascorbic acid, ergosterol, tocopherols, carotenoids), and minerals (zinc and selenium) [7]. Antioxidant agents scavenge oxidative species in the cell, thus reducing the levels of inflammation, which plays a huge role in many human diseases [11].
Pigments
The distinct reddish hue of the fly agaric cap (though it can also be yellow or orange) is the result of a complex mixture of pigments. Some of these pigments degrade swiftly, complicating their study. To date, chromatography experiments have fractionated the mixture into at least ten compounds. Muscaaurins generate an orange color, muscaflavin creates a yellow tone, muscapurpurin produces a reddish-violet hue, and muscarubrin is responsible for the red coloration [12].
Polysaccharides
Glucans, a type of polysaccharide, are abundant within fungal cell walls. Two studies have explored the potential anti-inflammatory and anti-tumoral activities of fucomannogalactan, a polysaccharide present in Amanita muscaria [13,14]. Still, more studies are needed to further characterize these molecules.
Izoxazoles
Izoxazoles are the most abundant compounds in fly agaric. Two of them are responsible for Amanita muscaria’s effects: ibotenic acid and agarin.
Ibotenic Acid
Ibotenic acid, also known as (S)-2-amino-2-(3-hydroxyisoxazol-5-yl)acetic acid, is a derivative of glutamic acid whose ionic form is known as glutamate. Glutamic acid is an α-amino acid used in protein synthesis across nearly all living organisms. Notably, it functions as the most important excitatory neurotransmitter in the mammalian central nervous system.
Glutamic acid is the precursor in the synthesis of γ-aminobutyric acid (GABA) in GABA-ergic neurons. Additionally, it acts as an uncompetitive agonist of N-methyl-D-aspartate (NMDA) receptors in multiple systems in the central nervous system. The NMDA receptors play a fundamental role in synaptic plasticity, the molecular mechanisms behind learning and memory [15]. They have potential roles in neurological conditions such as epilepsy, Parkinson’s, Alzheimer's, and Huntington’s disease [1,7,16].
Thanks to the structural similarities between glutamic acid and ibotenic acid, the latter acts as a non-selective NDMA receptor agonist [17]. As a result, it has strong neuronal excitatory properties and acts as a potent neurotoxin [18]. Ibotenic acid can induce seizures and lesions in specific brain areas, mirroring the lesions observed in Alzheimer’s disease. Remarkably, this specific neurotoxicity caused by ibotenic acid has facilitated its utilization in animals to develop Alzheimer’s disease models [16,19]. Together with agarin, ibotenic acid is responsible for the hallucinogenic effects of fly agaric. In humans, the dose of ibotenic acid to cause psychedelic effects ranges from 30 to 60 mg/kg [19]. Ibotenic acid is metabolized and converted into agarin through decarboxylation [20].
Agarin
Agarin (5-(aminomethyl)-isoxazole-3-ol) was isolated by three research groups during the 1960s [21,22]. It is a crystalline, colorless substance that dissolves readily in water, and is one of the primary components of Amanita muscaria. The compound is present in a very high concentration (0.03-0.1%) in fresh mushrooms [23]. Agarin, which can also be artificially synthesized in the laboratory [24], is an analog of the inhibitory neurotransmitter GABA, and thus, a selective agonist of the ionotropic GABA receptors. Remarkably, agarin acts as a potent GABA-A receptor agonist and a potent partial agonist at GABA-C receptors, while remaining inactive at GABA-B receptors [25-27]. It binds to these receptors in different regions of the forebrain, including the caudate nucleus and putamen, the thalamus, and the hippocampal formation.
When agarin binds to a GABA receptor, it prompts the opening of chloride ion channels, leading to the inhibition of neuronal activity in these areas [19]. In fact, agarin has been used as a treatment to inhibit hippocampal activity to demonstrate the importance of this brain region in generating long-term memory during sleep [28].
In a study conducted in rats, treatment with agarin increased both rapid eye movement and non-rapid eye movement stages during sleep, thus enhancing sleep duration and maintenance [29]. Agarin also decreased anxiety in a rat model when administered in the prelimbic cortex [30].
Anxiolytic effects were also observed in a model of chronic inflammatory pain, in this case when injected into the anterior cingulate cortex [31]. Agarin also prevented rat hepatocytes from liver injury induced by d-galactosamine as well as by alcohol [32,33].
These diverse effects of agarin are due to its ability to activate GABA receptors, as these molecules have positive effects in protecting the brain (neuroprotective properties), decreasing blood pressure and anxiety, and enhancing sleep quality [34,35]. To learn more about GABA’s molecular biology and beneficial effects, read this article.
Agarin toxicity has been evaluated across various species [36]. Its median lethal dose (LD50, or the dose lethal for 50% of tested subjects) is 22 mg/kg in mice (oral administration), 45 mg/kg in rats (oral administration), and 10 mg/kg in rabbits (intravenous administration). There is currently no available toxicology data for agarin alone in humans. Reports of mushroom intoxication linked to fly agaric have been reported in humans, however, this is infrequent due to the distinct appearance of Amanita muscaria, which sets it apart from other edible mushrooms.
Amanita muscaria: Poisoning Syndrome
Poisoning by Amanita muscaria is less severe compared to other species of the same genus, such as Amanita phalloides and Amanita pantherina, which are more hazardous. Agarin and ibotenic acid are rapidly absorbed by the gastrointestinal tract, becoming detectable in urine within 1 hour after ingestion. Both substances cross the blood-brain barrier to exert their effects on the central nervous system. Typical signs of Amanita muscaria poisoning, which are due to ibotenic acid in the mushroom, appear 30 minutes after consumption. The prevailing clinical symptoms encompass confusion, agitation, vomiting, somnolence, and hallucinations [37,38]. Generally, fly agaric does not induce organ damage, and intoxicated patients are not considered endangered unless there are concomitant treatments or medical conditions. Intoxication treatment is supportive and symptomatic, and gastric lavage or activated charcoal is only indicated within the first 2 hours after ingestion [7].
Conclusion
Mushrooms have been utilized by humans for nourishment and recreational practices for centuries. Siberian shamans incorporated Amanita muscaria in their rituals and ceremonies due to its hallucinogenic effects.
But it wasn’t easy to watch Santa Claus flying through the sky…
Due to the toxicity of ibotenic acid, shamans had to drink reindeer urine while other participants in ceremonies had to drink the urine of the shaman.
In contrast, agarin on its own does not have the same toxic properties as ibotenic acid and has profoundly positive effects on the GABA system as a GABA-A agonist. At high doses, it may also be hallucinogenic.
GABA deficiency syndrome is a very real and pervasive problem in the US, correlated with a host of mental health conditions such as anxiety, fear, depression, short temper, phobias, impulsiveness/disorganization, addictions (including alcoholism), schizophrenia, and OCD.
Could agarin help? Maybe Santa Claus knows. Does anyone know where he is?
Want to try agarin? Then check out Tro Zzz, our buccal troche formulated for sleep, which has agarin as one of its main ingredients!
References
-
Michelot D, Melendez-Howell LM. Amanita muscaria: chemistry, biology, toxicology, and ethnomycology. Mycol Res. 2003;107(Pt 2):131-146. doi:10.1017/s0953756203007305
-
Lee M, Dukan E, Milne I. Amanita Muscaria (Fly Agaric): From a Shamanistic Hallucinogen to the Search for Acetylcholine. J R Coll Physicians Edinb. 2018;48(1):85-91. doi:10.4997/jrcpe.2018.119
-
Main, Douglas. Magic Mushrooms May Explain Santa & His “Flying” Reindeer. LiveScience. Published December 20, 2012. https://www.livescience.com/25731-magic-mushrooms-santa-claus.html
-
Beres, Derek. Santa Claus was a psychedelic mushroom. Psychedelic Spotlight. Published December 23, 2020. https://psychedelicspotlight.com/santa-claus-was-a-psychedelic-mushroom/
-
Lumpert M, Kreft S. Catching flies with Amanita muscaria: traditional recipes from Slovenia and their efficacy in the extraction of ibotenic acid. J Ethnopharmacol. 2016;187:1-8. doi:10.1016/j.jep.2016.04.009
-
Yang ZL, Weiß M, Oberwinkler F. New species of Amanita from the eastern Himalaya and adjacent regions. Mycologia. 2004;96(3):636-646.
-
Voynova M, Shkondrov A, Kondeva-Burdina M, Krasteva I. Toxicological and pharmacological profile of Amanita muscaria (L.) Lam. – a new rising opportunity for biomedicine. Pharmacia. 2020;67(4):317-323. doi:10.3897/pharmacia.67.e56112
-
Lincoff, Gary. The Audubon Society Field Guide to North American Mushrooms. Knopf; 1981.
-
Tulloss, Rodham E. Amanita distribution in the Americas with comparison to eastern and southern Asia and notes on spore character variation with latitude and ecology. Mycotaxon. 2005;93:189-231.
-
Dunk CW, Lebel T, Keane PJ. Characterisation of ectomycorrhizal formation by the exotic fungus Amanita muscaria with Nothofagus cunninghamii in Victoria, Australia. Mycorrhiza. 2012;22(2):135-147. doi:10.1007/s00572-011-0388-9
-
Forman HJ, Zhang H. Targeting oxidative stress in disease: promise and limitations of antioxidant therapy. Nat Rev Drug Discov. 2021;20(9):689-709. doi:10.1038/s41573-021-00233-1
-
Stintzing F, Schliemann W. Pigments of Fly Agaric (Amanita muscaria). Z Für Naturforschung C. 2007;62(11-12):779-785. doi:10.1515/znc-2007-11-1201
-
Ruthes AC, Carbonero ER, Córdova MM, et al. Fucomannogalactan and glucan from mushroom Amanita muscaria: Structure and inflammatory pain inhibition. Carbohydr Polym. 2013;98(1):761-769. doi:10.1016/j.carbpol.2013.06.061
-
Alexandre SMA, Corradi da Silva ML, Vasconcelos AFD, et al. Rhizoctonia solani fucomannogalactan: Chemical characterization and antiproliferative activity. Int J Biol Macromol. 2018;115:106-113. doi:10.1016/j.ijbiomac.2018.04.035
-
Riedel G, Platt B, Micheau J. Glutamate receptor function in learning and memory. Behav Brain Res. 2003;140(1-2):1-47. doi:10.1016/s0166-4328(02)00272-3
-
Wang R, Reddy PH. Role of glutamate and NMDA receptors in Alzheimer’s disease. J Alzheimers Dis JAD. 2017;57(4):1041-1048. doi:10.3233/JAD-160763
-
Liljefors, Tommy L Tommy. Textbook of Drug Design and Discovery. 3rd ed. CRC Press; 2002.
-
Becker A, Grecksch G, Bernstein HG, Höllt V, Bogerts B. Social behaviour in rats lesioned with ibotenic acid in the hippocampus: quantitative and qualitative analysis. Psychopharmacology (Berl). 1999;144(4):333-338. doi:10.1007/s002130051015
-
Stebelska K. Fungal Hallucinogens Psilocin, Ibotenic Acid, and Muscimol: Analytical Methods and Biologic Activities. Ther Drug Monit. 2013;35(4):420. doi:10.1097/FTD.0b013e31828741a5
-
Nielsen EO, Schousboe A, Hansen SH, Krogsgaard-Larsen P. Excitatory amino acids: studies on the biochemical and chemical stability of ibotenic acid and related compounds. J Neurochem. 1985;45(3):725-731. doi:10.1111/j.1471-4159.1985.tb04052.x
-
Takemoto T, Nakajima T, Sakuma R. [ISOLATION OF A FLYCIDAL CONSTITUENT “IBOTENIC ACID” FROM AMANITA MUSCARIA AND A. PANTHERINA]. Yakugaku Zasshi. 1964;84:1233-1234.
-
Eugster CH, Müller GF, Good R. [The active ingredients from Amanita muscaria: ibotenic acid and muscazone]. Tetrahedron Lett. 1965;(23):1813-1815. doi:10.1016/s0040-4039(00)90133-3
-
DeFeudis FV. Physiological and behavioral studies with muscimol. Neurochem Res. 1980;5(10):1047-1068. doi:10.1007/BF00966163
-
Petersen JG, Bergmann R, Møller HA, et al. Synthesis and Biological Evaluation of 4-(Aminomethyl)-1-hydroxypyrazole Analogues of Muscimol as γ-Aminobutyric Acid A Receptor Agonists. J Med Chem. 2013;56(3):993-1006. doi:10.1021/jm301473k
-
Johnston GA, Kennedy SM, Lodge D. Muscimol uptake, release and binding in rat brain slices. J Neurochem. 1978;31(6):1519-1523. doi:10.1111/j.1471-4159.1978.tb06579.x
-
Krogsgaard-Larsen P, Johnston GA. Structure-activity studies on the inhibition of GABA binding to rat brain membranes by muscimol and related compounds. J Neurochem. 1978;30(6):1377-1382. doi:10.1111/j.1471-4159.1978.tb10469.x
-
Johnston GAR. Muscimol as an Ionotropic GABA Receptor Agonist. Neurochem Res. 2014;39(10):1942-1947. doi:10.1007/s11064-014-1245-y
-
Sawangjit A, Oyanedel CN, Niethard N, Salazar C, Born J, Inostroza M. The hippocampus is crucial for forming non-hippocampal long-term memory during sleep. Nature. 2018;564(7734):109-113. doi:10.1038/s41586-018-0716-8
-
Lancel M, Crönlein TAM, Faulhaber J. Role of GABAA Receptors in Sleep Regulation. Neuropsychopharmacology. 1996;15(1):63-74. doi:10.1016/0893-133X(95)00157-9
-
Green TA, Baracz SJ, Everett NA, Robinson KJ, Cornish JL. Differential effects of GABAA receptor activation in the prelimbic and orbitofrontal cortices on anxiety. Psychopharmacology (Berl). 2020;237(11):3237-3247. doi:10.1007/s00213-020-05606-9
-
Shao F bing, Fang J fan, Wang S si, et al. Anxiolytic effect of GABAergic neurons in the anterior cingulate cortex in a rat model of chronic inflammatory pain. Mol Brain. 2021;14:139. doi:10.1186/s13041-021-00849-9
-
Wang S, Xiang YY, Zhu J, et al. Protective roles of hepatic GABA signaling in acute liver injury of rats. Am J Physiol Gastrointest Liver Physiol. 2017;312(3):G208-G218. doi:10.1152/ajpgi.00344.2016
-
Wang S, Sui S, Liu Z, et al. Protective roles of hepatic gamma-aminobutyric acid signaling in acute ethanol exposure-induced liver injury. J Appl Toxicol JAT. 2018;38(3):341-350. doi:10.1002/jat.3544
-
Hepsomali P, Groeger JA, Nishihira J, Scholey A. Effects of Oral Gamma-Aminobutyric Acid (GABA) Administration on Stress and Sleep in Humans: A Systematic Review. Front Neurosci. 2020;14:923. doi:10.3389/fnins.2020.00923
-
Ngo DH, Vo TS. An Updated Review on Pharmaceutical Properties of Gamma-Aminobutyric Acid. Mol Basel Switz. 2019;24(15):2678. doi:10.3390/molecules24152678
-
Theobald W, Büch O, Kunz HA, Krupp P, Stenger EG, Heimann H. [Pharmacological and experimental psychological studies with 2 components of fly agaric (Amanita muscaria)]. Arzneimittelforschung. 1968;18(3):311-315.
-
Vendramin A, Brvar M. Amanita muscaria and Amanita pantherina poisoning: two syndromes. Toxicon Off J Int Soc Toxinology. 2014;90:269-272. doi:10.1016/j.toxicon.2014.08.067
-
Rampolli FI, Kamler P, Carnevale Carlino C, Bedussi F. The Deceptive Mushroom: Accidental Amanita muscaria Poisoning. Eur J Case Rep Intern Med. 2021;8(2):002212. doi:10.12890/2021_002212
Comments (0)
There are no comments for this article. Be the first one to leave a message!