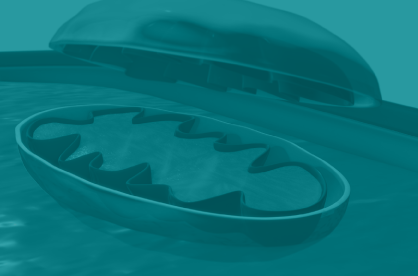
Think about the last time you felt exhausted after a full shift at work. Do you remember feeling foggy? Did you crave a meal loaded with carbs? Animals, plants, and fungi all need oxygen and a vast supply of carbon to keep going through the day.
In almost all eukaryotic cells, mitochondria run the cell’s metabolic machinery, transforming energy from food directly to energy. They produce impressive amounts of adenosine triphosphate (ATP), the bio-currency of cells, making use of oxygen and multiple enzymes attached to their inner membrane.
Depending on the energy needed by each cell, the number of mitochondria can vary. These organelles occupy almost half of your heart muscle cell and nearly 25% of the liver cell (approximately 2000 mitochondria per cell) [1]. They also participate in other equally crucial cell activities, including cell communication, lipid metabolism, immunity modulation, programmed cell death, and calcium homeostasis.
Unlike other cell organelles, mitochondria have two membranes outlining different internal spaces (matrix and intermembrane spaces) and their own DNA (mtDNA). This layer-type organization acts as a filter to control the flow of specific material. Combined, these compartments work relentlessly to provide energy in the form of ATP in a multi-step process.
What happens when this power machine malfunctions?
As expected, the heart, brain, and muscles are the parts of our body with high-energy demand and, therefore, are most affected by mitochondrial disease (MD). Several neurodegenerative diseases disorders such as Alzheimer’s, ALS, diabetes, heart disease, and even cancer are associated with mitochondrial dysfunction.
The cells’ power plant
ATP powers cellular processes via the chemical energy contained in its bonds. In oxygen-dependent organisms, the majority of ATP is produced in the mitochondria in its characteristic internal membrane folds, which create a very large surface area.
Mitochondrial membranes, like every other cell membrane, allow for the isolation of various ions and molecules. In the mitochondria, this membrane allows the creation of a “proton gradient force." Positively charged ions or protons concentrate only on one side of the membrane generating potential energy as an electrochemical gradient, similar to a battery with positive and negative ends. But in this case, protons can only cross the membrane via channel proteins that act as ion-loving transmembrane tunnels.
There are several essential proteins and molecules embedded in the inner mitochondrial membrane, comprising the electron transport chain (ETC). The ETC is a densely packed array of four enzyme complexes. Complexes I to IV operate as sort of turbines, producing energy mainly by a process called oxidative phosphorylation (OXPHOS), where electrons move from complex to complex and help create the proton gradient force.
Let’s get deep into the ETC!
When glucose and other carbon-based sugars are metabolized in the cell cytoplasm, electron carriers NADH and FADH2 transfer their electrons into the ETC to mark the start of OXPHOS. While NADH donates electrons to mitochondrial complex I, FADH2 can only feed them into complex II. Once the electron supply is delivered, they turn back to their oxidized forms, NAD+ and FAD+ molecules.
Indeed, electrons move through different redox centers in each enzyme complex depending on their affinity. The final redox center has an increased affinity for electrons compared to those at the beginning. This creates a consistent flow of electrons with the releasing of energy every time a movement is made from one center to another. This energy discharge helps complex I to pump protons, H+, across the inner membrane, from the inner mitochondrial matrix to the intermembrane space. Subsequently, this creates the proton gradient.
Electrons continue their journey, from complexes I and II to a transfer molecule named Coenzyme Q (CoQ), which is composed of lipids and is capable of running freely inside the membrane. Connecting multiple electron entry points, CoQ then delivers the electrons to complex III, which also uses liberated energy to pump H+ to the intermembrane space.
Similar to CoQ, a peripheral enzyme shuttle, cytochrome C carries electrons from complex III to IV. During this final step, complex IV forms water (H2O) using the received electrons, extra protons, and a molecule of oxygen (O2).
Yes, that's right, oxygen! The fundamental, molecular reason why we can't hold our breaths for too long or be without oxygen is that we need it as the final electron acceptor of the ETC. We must breathe and oxygenate our body and cells to keep energy production going.
When is ATP actually produced?
After the ETC, a built-up concentration of protons occurs in the mitochondrial intermembrane space. There is one tunnel protein that makes use of the proton gradient force to produce or synthesize ATP, and that is ATP synthase. This is the actual turbine in the power plant. Instead of water or air, the movement of protons through this channel-protein causes a change in the structure of the channel, catalyzing the addition of a phosphate group to the ATP precursor adenosine di-phosphate (ADP). Finally, energy in the form of ATP is made.
Of course, this is not an isolated event; like a power plant that never turns off its lights (well, almost never), this complex system is constantly working. For every glucose molecule that enters cellular respiration, approximately 30-34 ATP are generated [2], and this is replicated in every internal membrane of every mitochondrion in your body, fueling every cellular process.
How to Destroy or Enhance Your Mitochondrial Complexes
Several known molecules and even approved medicines can disrupt or enhance the function of the different ETC complexes. It is essential for those taking various medications or supplements (and those prescribing them) to understand their effects on the mitochondria.
- Destroy or Enhance Complex I and CoQ
Destroy with metformin and statins: Even the most conventionally prescribed medications can directly or indirectly cause mitochondrial dysfunction. Globally used for treating diabetes [2], metformin inhibits the activity of complex I and, as a result, decreases NADH, the proton gradient, and oxygen consumption. Patients with reduced kidney function, liver disease, or significant mitochondrial dysfunction need to be closely monitored when treated with these drugs to avoid lactic acidosis, which can develop if OXPHOS is inhibited [3].
Interestingly, however, metformin may also work as a hormetic stress, stimulating mitochondrial biogenesis. There have been several studies in patients with diabetes showing a longevity benefit, and its use has crept into the longevity space as well.
Statins, a group of medicines that help reduce LDL cholesterol, block the production of CoQ by inhibiting an intermediate enzyme. In rare cases, long-term treatment with statins can provoke muscle weakness (myopathy). How can this be mitigated? CoQ supplementation with your meals [4]!
Enhance with NAD precursors: The NADH cellular pool is the main OXPHOS rate determinator, and the balance between NAD+/NADH is controlled mainly by mitochondrial complex I. Fasting and exercising increase NAD+ supply. In contrast, overconsuming carbohydrates decreases NAD+ levels and correspondingly, NAD+ influx to the mitochondria decreases as well. NAD+ precursors such as nicotinic acid or niacin (A.K.A. vitamin B3) and nicotinamide (NAM) — long used in medicine to treat vitamin B deficiency — are effective in boosting mitochondrial metabolism in models of the heart muscle [5] and brain [6]. IV NAD+ has also become a popular treatment in the US and around the world, although research is still in its infancy.
- Destroy or Enhance Complex II
Destroy (Michael Jackson) with propofol: Bad joke, we know, but multiple anesthetics have shown mitochondrial toxicity at different doses. Especially interesting is the case of propofol, a sedative drug currently approved for use in more than 50 countries. Although the drug is safe, it can lead to the development of a severe syndrome that includes lactic acidosis and circulatory failure when used at high doses for prolonged periods of time. Propofol stops complex II and reduces cytochrome C levels. During the COVID-19 pandemic, patients requiring mechanical ventilation were usually sedated with this drug in intensive care units. In this context, several cases of propofol infusion syndrome were reported in recent years [7,8].
Enhance with B2: Vitamin B2 or riboflavin is an essential contributing factor to flavoproteins, enzymes present in both mitochondrial complexes I and II. Understandably, deficiency of vitamin B2 leads to ETC disruption and increased production of toxic reactive oxygen species (ROS) [9]. ROS production has multiple dramatic effects on the cell, including potential damage to mtDNA, which may lead to the malfunction of energy production, programmed cell death, and even reduction of lifespan. Controlling oxidative stress, a consequence of ROS, is essential. Interestingly, vitamin B2 cannot be naturally produced by the human body, so special bacteria in the large intestine produce free riboflavin. B2 is commonly found in eggs, milk, seafood, fortified cereals, and some vegetables. If you are a vegan or vegetarian athlete, pregnant, or breastfeeding, make sure to incorporate it in into your daily diet [10].
- Destroy or Enhance Complex III
Destroy with antimycin A: Complex III is the junction of the ETC, collecting electrons from both complexes I and II. Blocking complex III can drastically impact electron transfer in the ETC, increasing ROS generation. Antimycin A, a poison used to contain the fish population in fisheries, prevents the complex from accepting electrons and has been extensively used by researchers to study mitochondrial dysfunction.
Enhance with melatonin: Mitochondria contain high concentrations of melatonin compared to other subcellular compartments. There are actually special receptors that help mitochondria rapidly increase their melatonin uptake when needed. In addition to helping you sleep, melatonin acts as a ROS scavenger, maintaining optimal mitochondrial membrane potential and OXPHOS efficiency. It is no accident that, in the first hours of sleep, ATP levels surge in brain regions that were most active during the day [11].
As in complexes II and IV, iron (heme) groups are enclosed in the protein subunits of complex III and are crucial to its assembly as well as its ability to accept and donate electrons. Because of this, there is a close relationship between iron and OXPHOS. A recent study has shown that feeding extra iron to obese lab rats prevents body weight gain and fat accumulation in the liver. Moreover, iron promoted the expression of genes related to heme production in liver and muscle cells, thus regulating mitochondrial gene transcription and energy metabolism [12].
- Destroy or Enhance Complex IV
Destroy with carbon monoxide and cyanide: Carbon monoxide (CO) is found in some paint fumes, fires, and exhaust from cars or trucks. Gas or wooden stoves can leak CO as well. CO actively inhibits mitochondrial complex IV, and also tightly binds to hemoglobin molecules in red blood cells, shutting down OXPHOS and displacing oxygen, respectively. Unfortunately, this gas has no taste or odor and can initially cause headaches, dizziness, nausea, confusion, and even death with continued exposure.
Similarly, cyanide poisoning binds to complex IV, blocking the transfer of electrons to O2 and interfering with the proton pump function [13]. Please don’t try these at home, folks, even though there are potential reversal agents like methylene blue (see below).
Enhance with methylene blue: Methylene blue is a unique compound with the ability to interact and allow the free cycle flow of electrons in the ETC. Low-dose methylene blue stimulates OXPHOS since it serves as both donor and acceptor of electrons to complex IV and throughout the ETC. Methylene blue also helps create a metabolic cascade by creating a temporary low-oxygen local environment (due to increased O2 consumption by the ETC). This increases blood flow and glucose uptake in the brain, followed by a rise in complex IV and other mitochondrial expression levels in the neurons, creating a synergistic loop [14]. In the same way, photon absorption in complex IV after red light exposure also stimulates protein levels and energizes metabolism in the neurons via induction of complex activity [15]. Methylene blue is also a well-known antidote to cyanide poisoning and methemoglobinemia (CO poisoning).
Conclusion
Mitochondria are absolutely vital to maintaining and optimizing health. When they work well, we feel well, and when they don’t, we don’t. Symptoms of mitochondrial dysfunction can vary from fatigue and brain fog to the obliteration of the ETC from cyanide poisoning and death. Ouch!
Finally, it’s important to remember that the mitochondria require optimized cellular machinery, meaning that even if the complexes are functioning well, there is still a significant need for optimized levels of vitamins, minerals, and nutrients to ensure the Krebs cycle is humming along and donating electrons efficiently.
This is why it’s essential to not only measure mitochondrial dysfunction but to also assess the metabolites of the Krebs cycle.
Check out homehope.org for the framework that the physicians at Troscriptions use to detect and correct imbalances at the metabolomic level!
References
[1] Li A, Gao M, Jiang W, Qin Y, Gong G. Mitochondrial Dynamics in Adult Cardiomyocytes and Heart Diseases. Front Cell Dev Biol. 2020 Dec 17;8:584800. doi: 10.3389/fcell.2020.584800. PMID: 33392184; PMCID: PMC7773778.
[2] Atkinson, D.E. Cellular Energy Metabolism and Its Regulation. New York: Academic Press, 1977. p. 45.
[3] Vial G, Detaille D, Guigas B. Role of Mitochondria in the Mechanism(s) of Action of Metformin. Front Endocrinol (Lausanne). 2019 May 7;10:294. doi: 10.3389/fendo.2019.00294. PMID: 31133988; PMCID: PMC6514102.
[4] Qu H, Guo M, Chai H, Wang WT, Gao ZY, Shi DZ. Effects of Coenzyme Q10 on Statin-Induced Myopathy: An Updated Meta-Analysis of Randomized Controlled Trials. J Am Heart Assoc. 2018 Oct 2;7(19):e009835. doi: 10.1161/JAHA.118.009835. PMID: 30371340; PMCID: PMC6404871.
[5] O'Brien KD, Tian R. Boosting mitochondrial metabolism with dietary supplements in heart failure. Nat Rev Cardiol. 2021 Oct;18(10):685-686. doi: 10.1038/s41569-021-00610-8. PMID: 34404939; PMCID: PMC9172082.
[6] Lee CF, Caudal A, Abell L, Nagana Gowda GA, Tian R. Targeting NAD+ Metabolism as Interventions for Mitochondrial Disease. Sci Rep. 2019 Feb 28;9(1):3073. doi: 10.1038/s41598-019-39419-4. PMID: 30816177; PMCID: PMC6395802.
[7] Witenko CJ, Littlefield AJ, Abedian S, An A, Barie PS, Berger K. The Safety of Continuous Infusion Propofol in Mechanically Ventilated Adults With Coronavirus Disease 2019. Ann Pharmacother. 2022 Jan;56(1):5-15. doi: 10.1177/10600280211017315. Epub 2021 May 14. PMID: 33985368; PMCID: PMC8127019.
[8] Lucchetta V, Bonvicini D, Ballin A, Tiberio I. Propofol infusion syndrome in severe COVID-19. Br J Anaesth. 2020 Nov;125(5):e441-e442. doi: 10.1016/j.bja.2020.08.020. Epub 2020 Aug 24. PMID: 32912604; PMCID: PMC7444932.
[9] Bugiani M, Lamantea E, Invernizzi F, Moroni I, Bizzi A, Zeviani M, Uziel G. Effects of riboflavin in children with complex II deficiency. Brain Dev. 2006 Oct;28(9):576-81. doi: 10.1016/j.braindev.2006.04.001. Epub 2006 Jun 5. PMID: 16737791.
[10]. https://ods.od.nih.gov/factsheets/Riboflavin-HealthProfessional/
[11] Hardeland R. Melatonin and the electron transport chain. Cell Mol Life Sci. 2017 Nov;74(21):3883-3896. doi: 10.1007/s00018-017-2615-9. Epub 2017 Aug 7. PMID: 28785805.
[12] Kitamura N, Yokoyama Y, Taoka H, Nagano U, Hosoda S, Taworntawat T, Nakamura A, Ogawa Y, Tsubota K, Watanabe M. Iron supplementation regulates the progression of high fat diet induced obesity and hepatic steatosis via mitochondrial signaling pathways. Sci Rep. 2021 May 24;11(1):10753. doi: 10.1038/s41598-021-89673-8. PMID: 34031430; PMCID: PMC8144192.
[13] Mahernia S, Amanlou A, Kiaee G, Amanlou M. Determination of hydrogen cyanide concentration in mainstream smoke of tobacco products by polarography. J Environ Health Sci Eng. 2015 Jul 29;13:57. doi: 10.1186/s40201-015-0211-1. PMID: 26225214; PMCID: PMC4518591.
[14] Gonzalez-Lima F, Auchter A. Protection against neurodegeneration with low-dose methylene blue and near-infrared light. Front Cell Neurosci. 2015 May 12;9:179. doi: 10.3389/fncel.2015.00179. PMID: 26029050; PMCID: PMC4428125.
[15] Rojas JC, Gonzalez-Lima F. Low-level light therapy of the eye and brain. Eye Brain. 2011 Oct 14;3:49-67. doi: 10.2147/EB.S21391. PMID: 28539775; PMCID: PMC5436183.
Written by:
Estefanía Urdániz, PhD - Medical Writer
Edited by: Dr. Scott Sherr
Comments (0)
There are no comments for this article. Be the first one to leave a message!