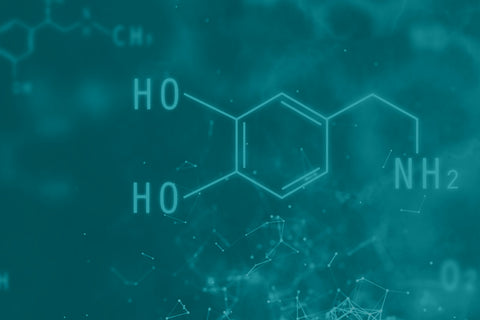
As with GABA or gamma-aminobutyric acid and serotonin, dopamine can’t cross the blood-brain barrier.
Although dopamine levels can generally be increased by consuming certain foods, the only way to pharmacologically increase dopamine in the brain is by using dopamine precursors, such as L-3,4-dihydroxyphenylalanine (L-dopa) [1]. This strategy has been used in the past to treat neurological disorders such as Parkinson’s disease, for example. And to prevent men from lactating (yes, it’s true… see below!).
Here, we will discuss the structure, function, and major roles of dopamine within the brain, central nervous system, and elsewhere + dabble in the dopaminergic mechanisms of certain classic psychedelics too.
What is Dopamine?
Dopamine, or 3,4-dihydroxyphenethylamine, has a well-known role in the brain as a neurotransmitter, which is a molecule that transmit signals from and between nerve cells (neurons).
It is involved in a wide range of important processes and has activity in the brain and central nervous system, as well as peripheral regions. In the brain, dopamine plays a role in reward and movement regulation, but it is also associated with the modulation of behavior and cognition, motivation, inhibition of prolactin production, sleep, dreaming, mood, attention, working memory, and learning [2].
The majority of dopamine synthesis occurs from the amino acid L-tyrosine to L-dopa (via the cofactor tyrosine hydroxylase), and then from L-dopa to dopamine (via aromatic L-amino acid decarboxylase) [2,3]. However, some dopamine can also be indirectly synthesized from the amino acid L-phenylalanine via the conversion of phenylalanine to tyrosine by phenylalanine hydroxylase [2].
The amino acid building blocks for dopamine (i.e., phenylalanine and tyrosine) are readily obtained from the diet, provided nutritional requirements for essential amino acids and/or protein are met. As previously mentioned, dopamine obtained from food or elsewhere cannot cross the blood-brain barrier and must therefore be synthesized within the brain.
The above "two-step" synthesis of dopamine takes place in the cytosol of dopaminergic neurons in the substantia nigra, ventral tegmental area, and hypothalamus in the brain [4], after which it is sequestered away into the lumen of synaptic vesicles. From here, dopamine is released when a nerve impulse traveling down the axon reaches the nerve terminal, and once in the synaptic cleft, it can act on postsynaptic and presynaptic receptors [3].
In adrenergic and noradrenergic cells, dopamine can also be further converted into norepinephrine and epinephrine through a process of sequential modification [2]. In terms of metabolism, dopamine is broken down in a sequential fashion by monoamine oxidase, catechol-O-methyl transferase, and aldehyde dehydrogenase [4]. Although there are several routes or pathways of dopamine degradation, the end-product is homovanillic acid (a metabolite with no known biological activity) that is subsequently excreted in the urine. This is a metabolite that can be measured as well on metabolomic testing (along with other neurotransmitter metabolites too!).
The History of Dopamine
Dopamine was first synthesized by George Barger and James Ewens in 1910 at the Wellcome Research Laboratories in England [3], and would later be described by Henry Dale as a weak sympathomimetic, epinephrine-like compound [5]. In 1952, Dale suggested its current name of dopamine.
Later, in 1957, Katharine Montagu was the first to discover dopamine in the brain of several species, including humans [6]. Building upon this work, Arvid Carlsson and Nils-Åke Hillarp at the University of Lund in Sweden revealed the role of dopamine as a neurotransmitter in a series of classic studies [7]. Carlsson himself would be awarded the Nobel Prize in Physiology or Medicine in 2000 for demonstrating that dopamine is more than just an intermediate in the formation of norepinephrine and epinephrine (i.e., noradrenaline and adrenaline), and is actually a neurotransmitter in its own right, with its own intrinsic roles and activities [3].
Dopamine Activity in the Brain, Nervous System, and Periphery
Dopamine exerts its activity by binding to cell surface receptors. In the brain, there are five target receptors, known as D1-5 receptors, and all belong to the large G-protein-coupled receptor family. These receptor subtypes are typically grouped into two major subclasses due to similarities in structure and drug sensitivity, forming the “D1-like” group (D1, D5) and “D2-like” group (D2, D3, D4), respectively [8].
The effect of dopamine on a target neuron largely depends on the types of receptors expressed on the neuronal membrane and the secondary effects within the neuron. For instance, D1-like activation of a neuron can be either excitatory (via the opening of sodium channels) or inhibitory (via the opening of potassium channels) [8]. Conversely, D2-like activation of a target neuron usually results in its inhibition.
Elsewhere in the body, dopamine has a variety of effects, such as the vasodilation of blood vessels, increased glomerular filtration rate in the kidneys, decreased insulin production in the pancreas, and decreased motility of the gastrointestinal tract [9]. In these areas, dopamine acts as a hormone.
How Dopamine Works
In the brain, there are four major pathways for dopaminergic activity. The first is the nigrostriatal pathway, wherein dopamine regulates the control of motor function and the acquisition of new motor skills [8].
The second dopaminergic pathway is the mesolimbic pathway, which is sometimes called the "reward" pathway. Here, mesolimbic dopamine released into the nucleus accumbens affects "incentive salience" (the motivation and desire for rewarding stimuli), and is therefore the pathway most implicated in addictive behavior [10]. It is this pathway that has indirectly garnered so much attention in popular culture, with some commentators suggesting that we live in a "dopamine-addicted" society.
The mesocortical pathway is associated with cognition and emotional behavior, with dopamine levels in the prefrontal cortex playing a key role in working memory and attention [8].
Lastly, the tuberoinfundibular pathway is involved in the secretion of prolactin, through which dopamine exerts an inhibitory effect.
Pharmacological Modulation of Dopamine
As with many neurotransmitters, the wide array of effects associated with dopamine has made it a major target for modulation with pharmacological compounds. Perhaps the most well-known of these is L-dopa, as described previously.
Parkinson’s disease is an aggressive and progressive neurodegenerative disorder that is associated with dopamine depletion in the central nervous system [9]. Arvid Carlsson, Oleh Hornykiewicz, and George Cotzias were instrumental in pioneering the development of levodopa (i.e., L-dopa) therapy for Parkinson’s disease patients. Even today, it is still the drug of choice to help manage, or control, symptoms of the disease [9] such as bradykinesia and rigidity. For decades, L-dopa has been used as a precursor to dopamine that can cross the blood-brain barrier and be readily converted to dopamine, thus temporarily diminishing the motor symptoms of the disease. Unfortunately, the long-term use of L-dopa is associated with adverse reactions in a majority of patients [9]. So much so that clinicians attempt to minimize its use by providing the medication only when absolutely necessary.
A separate example is the use of antipsychotic medications to treat conditions such as schizophrenia. The negative symptoms and cognitive deficits associated with the disorder might be driven by hypodopaminergic activity in the mesocortical pathway (see above) [11]. Antipsychotic drugs target dopamine transmission and are still the frontline therapeutic intervention for the disorder. They specifically target the dopamine D2 receptor as their primary site of action [11].
Similarly, a wide range of selective dopamine agonists or antagonists have been developed to treat nausea, erectile dysfunction, bipolar depression, hyperprolactinemia (which can occur in both men and women, and results in lactation/milk production!), and others by targeting the D2 receptor, such as apomorphine and bromopride [2].
The Influence of Psychedelics on Dopamine and its Receptors
Dopamine modulation and that of its receptors are controlled by several psychedelic compounds. Lysergic acid diethylamide (LSD or "acid"), a well-known psychedelic drug since the 1930s, acts upon D1 and D2 receptors as well as adrenergic receptors. Its activity at D2 receptors in animals has been linked with its behavioral effects but there is weaker evidence for this in human studies [12].
Psilocybin (contained in "magic mushrooms") has known effects on serotonin signaling but has also been found to increase striatal dopamine concentrations in humans, which correlate with feelings of euphoria and depersonalization [12]. However, psilocybin is not known to directly act upon dopamine receptors themselves.
Mescaline (3,4,5-trimethoxyphenethylamine) is a naturally occurring psychedelic compound (mainly found in the Peyote cactus) with similar effects to LSD and psilocybin. In cats, the behavioral effects of mescaline are driven by activity at both serotonin and dopamine receptors [13].
Finally, DMT (dimethyltryptamine) is a psychedelic most often associated with the ayahuasca brew prepared by many Amazonian tribes. Although its subjective effects are primarily mediated via serotonin receptors, it also indirectly alters the levels of dopamine, albeit without directly interacting with dopamine receptors themselves [14].
Conclusion
Dopamine has myriad essential functions both as a neurotransmitter and hormone. It gives us the incentive to keep being awesome… or compels us to continue watching cat videos. It is also the target of many pharmaceuticals and psychedelics. So enjoy it! But also do yourself a favor and consider a dopamine fast every once in a while. The best way, in our opinion, is to lock your phone up in a drawer for the day and get the f*ck outside in nature!
References
- R.M. Pinder, Possible Dopamine Derivatives capable of Crossing the Blood-Brain Barrier in relation to Parkinsonism, Nature. 228 (1970) 358–358. https://doi.org/10.1038/228358a0
- M.O. Klein, D.S. Battagello, A.R. Cardoso, D.N. Hauser, J.C. Bittencourt, R.G. Correa, Dopamine: Functions, Signaling, and Association with Neurological Diseases, Cell Mol Neurobiol. 39 (2019) 31–59. https://doi.org/10.1007/s10571-018-0632-3
- S. Fahn, The history of dopamine and levodopa in the treatment of Parkinson’s disease, Mov Disord. 23 Suppl 3 (2008) S497-508. https://doi.org/10.1002/mds.22028
- H. Juárez Olguín, D. Calderón Guzmán, E. Hernández García, G. Barragán Mejía, The Role of Dopamine and Its Dysfunction as a Consequence of Oxidative Stress, Oxid Med Cell Longev. 2016 (2016) 9730467. https://doi.org/10.1155/2016/9730467
- G. Barger, H.H. Dale, Chemical structure and sympathomimetic action of amines, J Physiol. 41 (1910) 19–59. https://doi.org/10.1113/jphysiol.1910.sp001392
- K.A. Montagu, Catechol compounds in rat tissues and in brains of different animals, Nature. 180 (1957) 244–245. https://doi.org/10.1038/180244a0
- F.M. Benes, Carlsson and the discovery of dopamine, Trends Pharmacol Sci. 22 (2001) 46–47. https://doi.org/10.1016/s0165-6147(00)01607-2
- G. Ayano, Dopamine: Receptors, Functions, Synthesis, Pathways, Locations and Mental Disorders: Review of Literatures, J Ment Disord Treat. 2 (2016). https://doi.org/10.4172/2471-271X.1000120
- F. Haddad, M. Sawalha, Y. Khawaja, A. Najjar, R. Karaman, Dopamine and Levodopa Prodrugs for the Treatment of Parkinson’s Disease, Molecules. 23 (2017) E40. https://doi.org/10.3390/molecules23010040
- K.C. Berridge, M.L. Kringelbach, Pleasure systems in the brain, Neuron. 86 (2015) 646–664. https://doi.org/10.1016/j.neuron.2015.02.018
- P. Li, G.L. Snyder, K.E. Vanover, Dopamine Targeting Drugs for the Treatment of Schizophrenia: Past, Present and Future, Curr Top Med Chem. 16 (2016) 3385–3403. https://doi.org/10.2174/1568026616666160608084834
- F.X. Vollenweider, K.H. Preller, Psychedelic drugs: neurobiology and potential for treatment of psychiatric disorders, Nat Rev Neurosci. 21 (2020) 611–624. https://doi.org/10.1038/s41583-020-0367-2
- R.J. Dinis-Oliveira, C.L. Pereira, D.D. da Silva, Pharmacokinetic and Pharmacodynamic Aspects of Peyote and Mescaline: Clinical and Forensic Repercussions, Curr Mol Pharmacol. 12 (2019) 184–194. https://doi.org/10.2174/1874467211666181010154139
- T.M. Carbonaro, M.B. Gatch, Neuropharmacology of N,N-dimethyltryptamine, Brain Res Bull. 126 (2016) 74–88. https://doi.org/10.1016/j.brainresbull.2016.04.016.
Comments (0)
There are no comments for this article. Be the first one to leave a message!