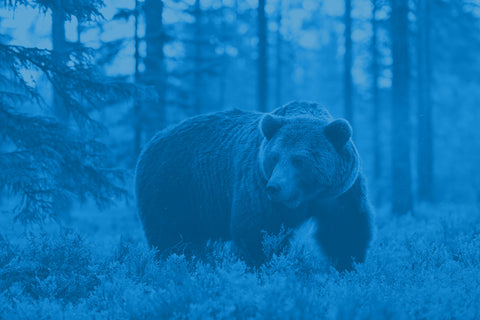
In today’s article, we are going to cover the “fight or flight” (or “oh sh*t, there’s a bear!”) catecholamine and neurotransmitter known as norepinephrine (NE).
As with gamma-aminobutyric acid (or GABA), serotonin, and dopamine, NE can’t cross the blood-brain barrier. Also called noradrenaline, NE mobilizes the brain and body for action in response to stressful or dangerous situations. The mechanisms, or systems, associated with NE action are the target of several pharmacological compounds, a selection of which will be explored in this article.
Here, we will discuss the structure, function, and key roles of NE within the brain and body, as well as its modulation by pharmacological and psychedelic drugs, heat, and cold thermogenesis.
What is Norepinephrine?
NE is involved in a variety of behavioral and physiological processes, including arousal, learning and memory, attention, mood, appetite, and in reaction to stress [1]. In the brain, it is one of the most abundant neurotransmitters [2], which are molecules that coordinate signals from and between neurons (nerve cells) and are associated with an array of diseases and conditions. Here, NE is produced by noradrenergic neurons in an area called the locus coeruleus [1]. Elsewhere in the body, it is produced in the adrenal medulla and postganglionic neurons of the sympathetic nervous system.
Synthesis of NE begins with the amino acid tyrosine, which can be obtained from the diet and also synthesized from the essential amino acid phenylalanine, which the body cannot produce itself. Rich sources of tyrosine include steak, pork, sockeye salmon, lean ground turkey, chicken, cheese, yogurt, and (for you vegans) tofu. Rich sources of phenylalanine include milk, eggs, cheese, nuts, soybeans, chicken, beef, pork, beans, and fish.
Tyrosine is converted into L-DOPA (levodopa) by the enzyme tyrosine hydroxylase, which is subsequently converted into dopamine by aromatic L-amino acid decarboxylase. Dopamine, another important catecholamine, is then converted into NE by dopamine beta-hydroxylase [3], therefore making dopamine a precursor of NE. Further conversion via phenylethanolamine N-methyl transferase produces epinephrine from NE in the adrenal glands (located on top of your kidneys) and selected regions of the brain.
As with many other neurotransmitters, NE cannot cross the blood-brain barrier and must therefore be synthesized within the brain from the amino acid building blocks consumed from protein in the diet (see above).
NE is involved in a range of important activities and processes, including wakefulness, arousal, attention, memory formation and consolidation, novelty-induced memory encoding, motivation, vigilance, reward, and addiction [4,5]. Most, if not all, of these roles are shared with the related neurotransmitter dopamine [4].
In terms of metabolism, NE is rapidly broken down by monoamine oxidase (MAO) or catechol-O-methyl transferase [3], and via several pathways the end-products are vanillylmandelic acid or 3-methoxy-4-hydroxyphenylglycol, two metabolites with no known biological activity that are subsequently excreted in urine.
The History of Norepinephrine
In 1914, Walter Cannon published the first scientific report of what he described as the “emergency response” of the body to life-threatening situations, stress, pain, and emotions such as fear and rage [6,7]. Later, in the early 1940s, the Swedish physiologist Ulf Svante von Euler first identified NE as a neurotransmitter, or impulse carrier (as it was called then), within the sympathetic nervous system [8]. He would later share the 1970 Nobel Prize in Physiology or Medicine with Julius Axelrod and Sir Bernard Katz.
Axelrod had discovered that the reuptake of NE into the nerve endings from which it was released represented a novel mechanism for neurotransmitter inactivation, and antidepressant drugs could act as inhibitors of this process [9]. He had also revealed the role of catechol-O-methyl transferase as an important enzyme in catecholamine metabolism.
Separately, Katz’s contribution concerned the quantal release of neurotransmitters – the way in which signals contained in electrical impulses travel along nerve fibers and are then relayed across gaps, or synapses, to other cells [10].
Collectively, the shared discoveries of these three scientists revealed the link between brain chemistry and psychiatric and nerve diseases, heralding in the search for psychoactive drugs [8,10].
Norepinephrine Activity in Neural Synapses
After its biosynthesis, NE is sequestered away in presynaptic vesicles. Following its release into the synaptic cleft, it exerts its effects through binding to the G-protein-coupled adrenergic receptors: α1A, α1B, α1D; α2A, α2B, α2C; or β1, β2, and β3 [11]. The α1 and β subtypes have a stimulatory effect on cell signaling, whereas the α2 subtype has an inhibitory effect on signaling. NE has the highest affinity for the α2 subtype of receptors, such that low-level release of the neurotransmitter may attenuate neuronal activity. Conversely, in the presence of high concentrations of NE, increased neural transmission occurs due to its binding to the stimulatory α1 and β receptors [11].
How Norepinephrine Works
During the characteristic “fight or flight” (or “oh sh*t, there’s a bear!") response, NE is released from the adrenal medulla and from sympathetic nerve terminals, causing a rise in circulatory concentrations of the hormone [12]. This increase in circulating NE gives rise to peripheral effects, such as increased blood pressure and cardiac output, relaxation of bronchial, intestinal, and other smooth muscles, pupil dilation, and alterations in metabolism that increase the availability of blood glucose and free fatty acids [12].
As mentioned above, the locus coeruleus is the major hub of NE in the brain, sending projections throughout the brain and also to the spinal cord. During sleep, activity in the locus coeruleus is very low and nearly negligible during REM (rapid eye movement) sleep [13]. Conversely, when presented with unpleasant stimuli, activity in this region increases and exhibits very high levels of activity in the presence of very stressful stimuli (such as intense fear of a bear, for example) [13].
Circulating concentrations of NE can also alter central aspects, such as memory for instance. This is a result of NE’s effects on afferent sensory nerves impacting central nervous system function [12]. NE also inhibits acute pain through α2-adrenergic receptors by pre-synaptic (inhibit neurotransmitters' release) and post-synaptic (hyperpolarize cell membranes) mechanisms.
Collectively, these physiological responses are necessary as a survival mechanism to preserve the life of the organism. However, prolonged elevation of circulating catecholamines can induce pathological conditions such as post-traumatic stress disorder, cardiac hypertrophy, hypertension, and heart failure [12].
Pharmacological Modulation of Norepinephrine
A number of highly important medications target NE systems throughout the body and brain.
Beta blockers (e.g., propranolol) are one such medication. These drugs antagonize the actions of NE at the previously discussed β-adrenergic receptor subtypes [14]. Although these drugs have been used to minimize the effects of anxiety in certain settings, such as performing arts (i.e., performing on stage or giving a speech without clothes on… just kidding) [15], their major application has been towards the long-term secondary prevention of heart attacks [16].
In contrast to beta blockers, alpha blockers target the α-adrenergic receptor subtypes and not the β subtypes, causing differing effects depending on the subtype. These drugs have been used for hypertension, in particular prazosin, doxazosin, and terazosin, due to their high affinity for α2 receptors [17]. These medications are also used for benign prostatic hypertrophy (BPH). Earlier, alpha blockers indiscriminately targeted α1 and α2 receptors and were therefore unsuitable for this purpose as they induced tachycardia and individuals developed a rapid tolerance to them [17].
MAO inhibitors (MAO-I) function as antidepressants by blocking the degradation of NE in addition to serotonin and dopamine [18]. Our favorite MAO-I is, of course, methylene blue (found in Just Blue and Blue Cannatine)… and that is why you don’t want to take methylene blue while on a SSRI or SNRI medication. More serotonin in the synapse could, theoretically, lead to serotonin syndrome which can be dangerous.
Effects of Psychedelics on Norepinephrine
A number of psychedelic drugs can be converted to catecholamines (such as NE) soon after ingestion, and these include mescaline (3,4,5-trimethoxyphenethylamine), psilocybin (“magic mushrooms”), lysergic acid diethylamide (LSD), and dimethyltryptamine (DMT) [19].
An early study from the late 1960s found that LSD and psilocybin both reduced the abundance of NE in the rat hypothalamus [20]. Both drugs impeded the acquisition of a conditioned avoidance response, with the amounts necessary to elicit gross behavioral excitation equivalent to the dose required to deplete NE.
Another study from the same time period found that phencyclidine (PCP), LSD, and mescaline all decreased levels of NE and increased dopamine slightly [21]. The authors proposed that hallucinogenic drugs such as these block the release of NE from the neuron, allowing its breakdown by MAO.
Lastly, a study from 1980 observed that LSD, psilocybin, and mescaline had no effect on the glutamate-induced excitation of facial motoneurons by themselves. However, a major conclusion was that these psychedelics potentiated, or primed, the effect of monoamines on facial motoneurons by sensitizing serotonin and NE receptors [22].
Temperature Fluctuations and the Release of Norepinephrine
Cold-induced thermogenesis is the ability to generate heat by increasing metabolism in response to cold ambient temperatures to facilitate a stable overall body temperature [23]. In humans, cold stress increases plasma concentrations of NE [24], which brings about an increase in lipolysis (or fat breakdown), suggesting a mobilization of fat stores to deal with the substantial physiological stress. Interestingly, older people have a reduced ability to mobilize fat stores in this manner, as shown by lower plasma NE concentrations, reduced vasoconstriction, and lower core body temperature [25].
On the other hand, NE levels in the brains (preoptic area and anterior hypothalamus) of rats have also been shown to increase in response to varying heat exposures (30-45 °C) [26]. Elsewhere in humans, 30 minutes of whole-body heat stress (73 °C in a dry heat sauna) was shown to increase plasma NE by 58% in young healthy men and women [27]. These data highlight the important role of NE as a regulator of blood pressure, homeostatic control, and thermoregulation in response to thermal strain.
Conclusion
NE is made from dopamine, which is made from the amino acid tyrosine. It plays many different roles systemically and as a neurotransmitter, however, all of its roles are focused on inducing the body into the “fight or flight” or “oh sh*t, there is a lion, tiger, or bear” response. The end result? You will move faster, think faster, focus, and feel less pain. If you’ve ever injured a body part (hopefully not that body part… ouch!) and felt no pain for a little while, this was because your body/brain was flooded with NE!
Too much NE or having it stick around for too long, however, can be quite detrimental to your health. This is why it’s essential to have a balance between your NE response and the responses of the neurotransmitters and hormones of your parasympathetic nervous system too. More on that in a later blog article!
References
- E. Saboory, M. Ghasemi, N. Mehranfard, Norepinephrine, neurodevelopment and behavior, Neurochemistry International. 135 (2020) 104706. https://doi.org/10.1016/j.neuint.2020.104706
- D. Weinshenker, J.P. Schroeder, There and Back Again: A Tale of Norepinephrine and Drug Addiction, Neuropsychopharmacol. 32 (2007) 1433–1451. https://doi.org/10.1038/sj.npp.1301263
- I.J. Kopin, Biosynthesis and Metabolism of Catecholamines, Anesthesiology. 29 (1968) 654–660. https://doi.org/10.1097/00000542-196807000-00007
- Y. Ranjbar-Slamloo, Z. Fazlali, Dopamine and Noradrenaline in the Brain; Overlapping or Dissociate Functions?, Front. Mol. Neurosci. 12 (2020) 334. https://doi.org/10.3389/fnmol.2019.00334
- I. Prokopová, Noradrenaline and behavior, Cesk Fysiol. 59 (2010) 51–58.
- W.B. Cannon, The Emergency Function of the Adrenal Medulla in Pain and the Major Emotions, American Journal of Physiology-Legacy Content. 33 (1914) 356–372. https://doi.org/10.1152/ajplegacy.1914.33.2.356
- J.A. Ross, E.J. Van Bockstaele, The Locus Coeruleus- Norepinephrine System in Stress and Arousal: Unraveling Historical, Current, and Future Perspectives, Front Psychiatry. 11 (2020) 601519. https://doi.org/10.3389/fpsyt.2020.601519
- M.A. Shampo, R.A. Kyle, Ulf von Euler--norepinephrine and the Nobel Prize, Mayo Clin Proc. 70 (1995) 273. https://doi.org/10.4065/70.3.273
- L. Iversen, Julius Axelrod: 20 May 1912 - 29 December 2004, Biogr Mem Fellows R Soc. 52 (2006) 1–13. https://doi.org/10.1098/rsbm.2006.0002
- P. Wright, Sir Bernard Katz, The Lancet. 362 (2003) 85. https://doi.org/10.1016/S0140-6736(03)13835-4
- V. Maletic, A. Eramo, K. Gwin, S.J. Offord, R.A. Duffy, The Role of Norepinephrine and Its α-Adrenergic Receptors in the Pathophysiology and Treatment of Major Depressive Disorder and Schizophrenia: A Systematic Review, Front. Psychiatry. 8 (2017). https://doi.org/10.3389/fpsyt.2017.00042
- A. William Tank, D. Lee Wong, Peripheral and Central Effects of Circulating Catecholamines, Comprehensive Physiology. (2014) 1–15. https://doi.org/10.1002/cphy.c140007
- C.W. Berridge, B.E. Schmeichel, R.A. España, Noradrenergic modulation of wakefulness/arousal, Sleep Med Rev. 16 (2012) 187–197. https://doi.org/10.1016/j.smrv.2011.12.003
- A.J. Wood, Pharmacologic differences between beta blockers, Am Heart J. 108 (1984) 1070–1077. https://doi.org/10.1016/0002-8703(84)90583-0
- K.A. Neftel, R.H. Adler, L. Käppeli, M. Rossi, M. Dolder, H.E. Käser, H.H. Bruggesser, H. Vorkauf, Stage fright in musicians: a model illustrating the effect of beta blockers, Psychosom Med. 44 (1982) 461–469. https://doi.org/10.1097/00006842-198211000-00008
- N. Freemantle, J. Cleland, P. Young, J. Mason, J. Harrison, Beta blockade after myocardial infarction: systematic review and meta regression analysis, BMJ. 318 (1999) 1730–1737. https://doi.org/10.1136/bmj.318.7200.1730
- D.T. Nash, Alpha-adrenergic blockers: mechanism of action, blood pressure control, and effects of lipoprotein metabolism, Clin Cardiol. 13 (1990) 764–772. https://doi.org/10.1002/clc.4960131104
- J.P.M. Finberg, J.M. Rabey, Inhibitors of MAO-A and MAO-B in Psychiatry and Neurology, Front Pharmacol. 7 (2016) 340. https://doi.org/10.3389/fphar.2016.00340
- P.J. Fitzgerald, Many Drugs of Abuse May Be Acutely Transformed to Dopamine, Norepinephrine and Epinephrine In Vivo, IJMS. 22 (2021) 10706. https://doi.org/10.3390/ijms221910706
- M.F. Sugrue, A study of the role of noradrenaline in behavioral changes produced in the rat by psychotomimetic drugs, Br J Pharmacol. 35 (1969) 243–252. https://doi.org/10.1111/j.1476-5381.1969.tb07983.x
- B.E. Leonard, S.R. Tonge, The effects of some hallucinogenic drugs upon the metabolism of noradrenaline, Life Sciences. 8 (1969) 815–825. https://doi.org/10.1016/0024-3205(69)90142-8
- R.B. McCall, G.K. Aghajanian, Hallucinogens potentiate responses to serotonin and norepinephrine in the facial motor nucleus, Life Sciences. 26 (1980) 1149–1156. https://doi.org/10.1016/0024-3205(80)90654-2
- R.J. Brychta, K.Y. Chen, Cold-induced thermogenesis in humans, Eur J Clin Nutr. 71 (2017) 345–352. https://doi.org/10.1038/ejcn.2016.223
- K. Nishiyama, Y.-I. Kamijo, J.W. van der Scheer, T. Kinoshita, V.L. Goosey-Tolfrey, S.P. Hoekstra, Y. Nishimura, T. Kawasaki, T. Ogawa, F. Tajima, Lipid metabolism after mild cold stress in persons with a cervical spinal cord injury, Spinal Cord. (2022). https://doi.org/10.1038/s41393-022-00788-9
- S.M. Frank, S.N. Raja, C. Bulcao, D.S. Goldstein, Age-related thermoregulatory differences during core cooling in humans, Am J Physiol Regul Integr Comp Physiol. 279 (2000) R349-354. https://doi.org/10.1152/ajpregu.2000.279.1.R349
- H. Nakagawa, T. Ishiwata, Effect of short- and long-term heat exposure on brain monoamines and emotional behavior in mice and rats, Journal of Thermal Biology. 99 (2021) 102923. https://doi.org/10.1016/j.jtherbio.2021.102923
- M. Iguchi, A.E. Littmann, S.-H. Chang, L.A. Wester, J.S. Knipper, R.K. Shields, Heat stress and cardiovascular, hormonal, and heat shock proteins in humans, J Athl Train. 47 (2012) 184–190. https://doi.org/10.4085/1062-6050-47.2.184
Comments (0)
There are no comments for this article. Be the first one to leave a message!