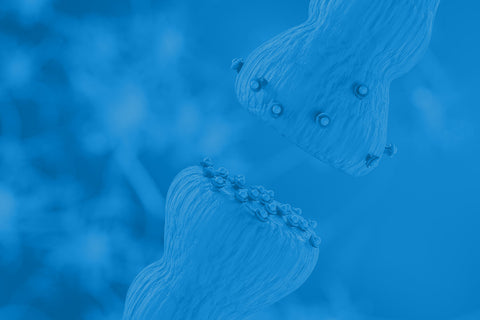
Get out your eggs! In this article, we will explore an important neurotransmitter in the body and brain called acetylcholine (ACh).
While also functioning as a classical neuromodulator, ACh is similar to norepinephrine, serotonin, and dopamine in that it cannot cross the blood-brain barrier.
It is an essential neurotransmitter that exerts its effects at the neuromuscular junction – the interface between a motor neuron and a muscle fiber – and is therefore integral for muscle activation.
In this article, we will discuss the structure, function, and key roles and effects of ACh within the brain and body, as well as its modulation by pharmacological and psychedelic drugs. We will also discuss how nicotine and methylene blue interact with ACh… because those compounds are of special interest to us. :)
What is Acetylcholine?
ACh is a neurotransmitter involved in the process of signal transduction from a nerve impulse to the muscle fiber, bringing about muscle contraction. It acts within the central (i.e., brain and spinal cord) and peripheral (i.e., other nerves and ganglia) nervous systems. Bodily systems that are affected by acetylcholine are called cholinergic systems, with compounds that affect such systems referred to as cholinergics (increase activity) and anticholinergics (decrease activity).
In the central nervous system, ACh influences cognitive function, alertness, memory, and learning; whereas in the autonomic nervous system (sympathetic and parasympathetic), ACh is used to generate a nerve impulse [1].
The synthesis of ACh involves acetyl coenzyme-A, choline, and the unique ACh biosynthetic enzyme choline acetyltransferase (ChAT) [2]. Without ChAT, motor neurons are unable to activate muscle fibers at their neuromuscular junctions. Choline is produced within the body but generally not to a sufficient degree – neurons cannot synthesize it and it is therefore obtained from the diet and delivered by the bloodstream [2]. Dietary sources of choline include foods such as meat and eggs. However, choline intakes in the United States are far below adequate levels [3].
Acetylcholinesterase is an enzyme that catalyzes (i.e., speeds up) the breakdown of ACh and serves as the major cholinesterase in the body. ACh is catalyzed by acetylcholinesterase into inactive metabolites (choline and acetate) that are subsequently reused or excreted. Almost 50% of the choline derived from ACh breakdown is recovered by high-affinity choline transporters [2].
As with many other neurotransmitters, ACh cannot cross the blood-brain barrier and must be synthesized in the brain and elsewhere to exert its effects.
The History of Acetylcholine
In 1867, the German chemist Adolf von Baeyer chemically synthesized ACh, but its biological activity would not be unraveled for at least another four decades [4]. Indeed, around one hundred years ago the concept of neurotransmitters was still in its infancy. In 1914, Sir Henry Hallett Dale and his colleague Arthur J. Ewins were the first to discover ACh in a natural organism after extracting it from ergot fungus [4], an event claimed by Dale as a "lucky accident" [5]. Side note: we wonder what kind of “fungus” they were also sampling at the same time as this “lucky accident!"
Shortly thereafter in 1921, the Austrian pharmacologist and psychobiologist Otto Loewi provided definitive physiological evidence for chemical transmission of vagus nerve impulses in a classic series of frog experiments that would later be attributed to ACh in 1926 [4]. Dale and Harold Dudley would successfully extract and chemically identify ACh as a natural constituent of the mammalian body in 1929 [4], continuing to publish seminal papers (24 in total) on the topic throughout the early to mid-1930s [5].
For their combined efforts on the chemical transmission of nerve impulses, Dale and Loewi would both share the 1936 Nobel Prize in Physiology or Medicine.
How Acetylcholine and Cholinergic Receptors Work
ACh receptors are expressed almost ubiquitously, ranging from neuromuscular junctions and the parasympathetic nervous system to cortical regions in the brain that regulate memory, learning, and cognition [6]. ACh action is mediated by binding to two different membrane-bound receptor classes, or subtypes, as follows: (1) G‑protein-coupled muscarinic acetylcholine receptors, and (2) ligand-gated nicotinic acetylcholine receptors [7].
Nicotinic and muscarinic ACh receptors are localized both pre- and post-synaptically. Pre-synaptic muscarinic ACh receptors are mainly inhibitory, whereas post-synaptic muscarinic ACh receptors can be either inhibitory or excitatory.
Pre-synaptic nicotinic ACh receptors stimulate the release of an array of neurotransmitters, including GABA, glutamate, dopamine, serotonin, norepinephrine, and ACh. Their post-synaptic counterparts depolarize neurons, increase their firing rate, and contribute to long-term potentiation [8]. Can you guess which one nicotine stimulates? See below!
The autoimmune neurological disorder myasthenia gravis is characterized by the manifestation of antibodies that act against the ACh receptor, muscle-specific kinase, or other ACh-associated proteins in the post-synaptic membrane [9]. These antibodies block or disrupt cholinergic transmission between nerve terminals and muscle fibers by downregulating, destroying, or functionally blocking ACh receptors in the post-synaptic space, with symptoms manifesting as varying degrees of muscle weakness [10]. Management of the condition typically includes anticholinesterase inhibitors (see below) as part of the overall regime or addressing the underlying etiology of the condition (such as in a paraneoplastic syndrome from cancer).
Vascular Effects of Acetylcholine Release
The endothelium lines the heart and blood vessels and plays a fundamental role in the vascular response to chemical and mechanical stimuli. Endothelial cells are particularly sensitive to ACh, which is released by the endothelium in response to blood flow. Once ACh is liberated in intact arteries, it triggers the release of calcium from an internal store in endothelial cells, causing the production of nitric oxide and subsequent relaxation and dilation of the blood vessel [11]. These findings are also corroborated by previous work from the early 2000s which found that the exogenous application of ACh to human skin induces vasodilation, primarily due to nitric oxide and prostaglandin production [12]. Therefore, in addition to its role as a neurotransmitter, ACh appears to be an important intermediary in the vascular response to blood flow.
The Role of Acetylcholine in Memory
The effects of ACh on memory and cognitive function are somewhat complex. The modulation of cognitive function by ACh appears to be selective to hippocampus-dependent memory, affecting spatial but not procedural memory [13]. There are also differential effects depending on the receptor subtype (i.e., muscarinic or nicotinic) that is targeted by ACh. Both receptor subtypes are involved in the encoding of new memories, as revealed by pharmacological data, with ACh acting as a potentiating factor in the encoding process [14]. The ability of ACh to enhance memory function is also contingent on the baseline level of cholinergic activity – it seems to have a much more prominent effect in those with low ACh tone [13].
Pharmacological Modulation of Acetylcholine
A number of highly important medications target cholinergic systems. Given the influence of ACh on muscle activity, the pharmacological modulation of this neurotransmitter can be potentially dangerous, yet this has potential uses in medicine and in nature.
Botulinum toxin (Botox) is a well-known neurotoxic protein produced by the bacterium Clostridium botulinum that is used in cosmetics and other applications, including for migraine headaches. All Botox serotypes (of which there are eight) interfere with neural transmission by blocking the release of ACh at the neuromuscular junction, causing muscle paralysis [15]. Botox therefore has notable applications for hemifacial spasm, dystonia, headaches, temporomandibular joint problems, and other chronic disorders involving muscle contraction and function.
In nature, the famous black widow spiders (Latrodectus) possess neurotoxic venom (alpha-latrotoxin) that brings about the depletion of ACh, the exhaustion of which causes paralysis. In a similar way, the indigenous peoples of Central and South America use alkaloid arrow poisons (curare) for paralyzing purposes during hunting [16]. Such poisons competitively and reversibly bind to nicotinic ACh receptors at the neuromuscular junction, causing skeletal muscle weakness and eventual death due to asphyxiation. As the nature of this binding is reversible, acetylcholinesterase inhibitors can be used to restore motor neuron function and muscle movement in the event of curare poisoning.
Certain medical settings require the administration of muscle relaxants, of which there are nondepolarizing varieties that act as competitive antagonists of ACh at nicotinic cholinergic receptors within the neuromuscular junction in skeletal muscle [17]. Once the medical procedure in question is finished (such as intubation for anesthesia), it is necessary to reverse the neuromuscular block, and this is accomplished using anticholinesterase drugs, which decrease the metabolism of ACh at the neuromuscular junction and allow its concentration to increase and overcome the action of the muscle relaxant [17].
Effects of Psychedelics on Acetylcholine
Evidence on the effects of psychedelics on ACh is limited, though a couple of compounds appear to be capable of modulating ACh. Early research from the 1970s suggests that dimethyltryptamine (DMT) significantly decreases ACh in the corpus striatum (a part of the basal ganglia), potentially due to a greater rate of ACh release or turnover [18]. Interestingly, DMT had no effect on the concentrations of ACh in the cortex.
In a study from the mid-1980s, lysergic acid diethylamide (LSD) was found to stimulate serotonin receptors while decreasing evoked ACh release in smooth muscle from guinea pigs, also bringing about increased muscle tone [19].
Nicotine and Acetylcholine Receptors
Nicotine acts like ACh in that it is a nicotinic receptor agonist – it causes a conformational change that either opens or closes the ion channels of the receptor, thus changing its functional state [20]. This binding augments the release of numerous neurotransmitters such as dopamine, serotonin, norepinephrine, ACh, GABA, and glutamate [21].
When vaped, smoked, or used at high doses orally, nicotine administration can modify the plasticity within the cholinergic system, particularly in the midbrain, bringing about nicotine dependence [22]. Partial agonists of these receptors have garnered attention as a means to enhance the likelihood of quitting smoking, but drug targets need further refinement.
However, at low doses and when not smoked or vaped, nicotine is a fantastic nootropic with a well-known safety profile due to its release of these neurotransmitters, especially ACh. It has been studied and shown to be effective in improving memory, recall, verbal fluidity, and focus.
Blue Cannatine, our flagship product at Troscriptions, contains low-dose (1 mg/troche) tobacco-free pharmaceutical grade nicotine in combination with caffeine, cannabidiol, and methylene blue. For 3 to 5 hours, you’ll launch your focus, productivity, verbal fluidity, energy, and flow.
Speaking of methylene blue…
How are Acetylcholine Receptors Affected by Methylene Blue?
Methylene blue (methylthionine chloride) was first introduced in the 19th century and has found several applications in medicine and biology [23]. Research from the late 1990s suggests that methylene blue is a cholinesterase inhibitor with a preference for muscarine ACh receptors [24], and thus increases the availability of ACh. This quality is shared with other cholinesterase inhibitors that are used as long-term symptomatic treatments for Alzheimer’s disease [23]. This may be one of the major reasons why methylene blue improves focus and memory.
References
[1] S.F. Kokaz, P.K. Deb, S.N. Abed, A. Al-Aboudi, N. Das, F.A. Younes, R.A. Salou, Y.A. Bataineh, K.N. Venugopala, R.P. Mailavaram, Pharmacology of Acetylcholine and Cholinergic Receptors, in: P. Kumar, P.K. Deb (Eds.), Frontiers in Pharmacology of Neurotransmitters, Springer Singapore, Singapore, 2020: pp. 69–105. https://doi.org/10.1007/978-981-15-3556-7_3.
[2] F. Amenta, S.K. Tayebati, Pathways of acetylcholine synthesis, transport and release as targets for treatment of adult-onset cognitive dysfunction, Curr Med Chem. 15 (2008) 488–498. https://doi.org/10.2174/092986708783503203.
[3] S.H. Zeisel, K.-A. da Costa, Choline: an essential nutrient for public health, Nutr Rev. 67 (2009) 615–623. https://doi.org/10.1111/j.1753-4887.2009.00246.x.
[4] K. Kawashima, T. Fujii, Y. Moriwaki, H. Misawa, K. Horiguchi, Non-neuronal cholinergic system in regulation of immune function with a focus on α7 nAChRs, International Immunopharmacology. 29 (2015) 127–134. https://doi.org/10.1016/j.intimp.2015.04.015.
[5] E.M. Tansey, Henry Dale and the discovery of acetylcholine, C R Biol. 329 (2006) 419–425. https://doi.org/10.1016/j.crvi.2006.03.012.
[6] J. Svoboda, A. Popelikova, A. Stuchlik, Drugs Interfering with Muscarinic Acetylcholine Receptors and Their Effects on Place Navigation, Front. Psychiatry. 8 (2017) 215. https://doi.org/10.3389/fpsyt.2017.00215.
[7] D. Bertrand, T.L. Wallace, A Review of the Cholinergic System and Therapeutic Approaches to Treat Brain Disorders, Curr Top Behav Neurosci. 45 (2020) 1–28. https://doi.org/10.1007/7854_2020_141.
[8] M.R. Picciotto, M.J. Higley, Y.S. Mineur, Acetylcholine as a neuromodulator: cholinergic signaling shapes nervous system function and behavior, Neuron. 76 (2012) 116–129. https://doi.org/10.1016/j.neuron.2012.08.036.
[9] N.E. Gilhus, S. Tzartos, A. Evoli, J. Palace, T.M. Burns, J.J.G.M. Verschuuren, Myasthenia gravis, Nat Rev Dis Primers. 5 (2019) 30. https://doi.org/10.1038/s41572-019-0079-y.
[10] L. Dresser, R. Wlodarski, K. Rezania, B. Soliven, Myasthenia Gravis: Epidemiology, Pathophysiology and Clinical Manifestations, J Clin Med. 10 (2021) 2235. https://doi.org/10.3390/jcm10112235.
[11] C. Wilson, M.D. Lee, J.G. McCarron, Acetylcholine released by endothelial cells facilitates flow-mediated dilatation, J Physiol. 594 (2016) 7267–7307. https://doi.org/10.1113/JP272927.
[12] D.L. Kellogg, J.L. Zhao, U. Coey, J.V. Green, Acetylcholine-induced vasodilation is mediated by nitric oxide and prostaglandins in human skin, Journal of Applied Physiology. 98 (2005) 629–632. https://doi.org/10.1152/japplphysiol.00728.2004.
[13] J. Haam, J.L. Yakel, Cholinergic modulation of the hippocampal region and memory function, J Neurochem. 142 Suppl 2 (2017) 111–121. https://doi.org/10.1111/jnc.14052.
[14] M.E. Hasselmo, The role of acetylcholine in learning and memory, Curr Opin Neurobiol. 16 (2006) 710–715. https://doi.org/10.1016/j.conb.2006.09.002.
[15] P.K. Nigam, A. Nigam, Botulinum toxin, Indian J Dermatol. 55 (2010) 8–14. https://doi.org/10.4103/0019-5154.60343.
[16] M.M. Rahman, T. Basta, J. Teng, M. Lee, B.T. Worrell, M.H.B. Stowell, R.E. Hibbs, Structural mechanism of muscle nicotinic receptor desensitization and block by curare, Nat Struct Mol Biol. 29 (2022) 386–394. https://doi.org/10.1038/s41594-022-00737-3.
[17] J.E. Caldwell, Clinical limitations of acetylcholinesterase antagonists, J Crit Care. 24 (2009) 21–28. https://doi.org/10.1016/j.jcrc.2008.08.003.
[18] T.M. Carbonaro, M.B. Gatch, Neuropharmacology of N,N-dimethyltryptamine, Brain Res Bull. 126 (2016) 74–88. https://doi.org/10.1016/j.brainresbull.2016.04.016.
[19] I. Pfeuffer-Friederich, H. Kilbinger, The effects of LSD in the guinea-pig ileum. Inhibition of acetylcholine release and stimulation of smooth muscle, Naunyn Schmiedebergs Arch Pharmacol. 331 (1985) 311–315. https://doi.org/10.1007/BF00500812.
[20] A.P. Govind, P. Vezina, W.N. Green, Nicotine-induced upregulation of nicotinic receptors: underlying mechanisms and relevance to nicotine addiction, Biochem Pharmacol. 78 (2009) 756–765. https://doi.org/10.1016/j.bcp.2009.06.011.
[21] E. Quattrocki, A. Baird, D. Yurgelun-Todd, Biological aspects of the link between smoking and depression, Harv Rev Psychiatry. 8 (2000) 99–110.
[22] C. Xiao, C. Zhou, J. Jiang, C. Yin, Neural circuits and nicotinic acetylcholine receptors mediate the cholinergic regulation of midbrain dopaminergic neurons and nicotine dependence, Acta Pharmacol Sin. 41 (2020) 1–9. https://doi.org/10.1038/s41401-019-0299-4.
[23] M. Oz, D.E. Lorke, M. Hasan, G.A. Petroianu, Cellular and molecular actions of Methylene Blue in the nervous system, Med Res Rev. 31 (2011) 93–117. https://doi.org/10.1002/med.20177.
[24] M. Pfaffendorf, T.A. Bruning, H.D. Batnik, P.A. van Zwieten, The interaction between methylene blue and the cholinergic system, Br J Pharmacol. 122 (1997) 95–98. https://doi.org/10.1038/sj.bjp.0701355.
Comments (0)
There are no comments for this article. Be the first one to leave a message!