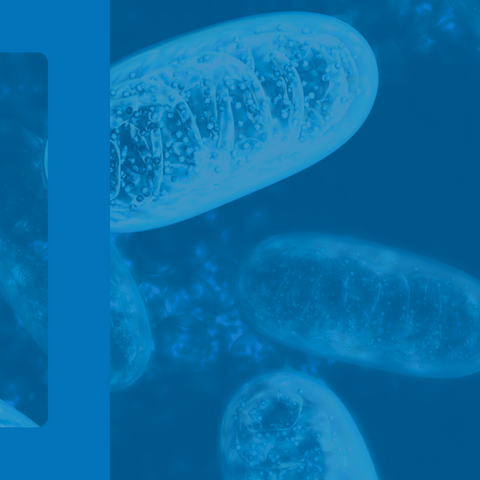
Mitochondria are responsible for generating more than 90% of the body's energy, primarily in the form of adenosine triphosphate (ATP) [1]. When ATP breaks down, it releases essential energy crucial for maintaining life and ensuring proper functioning of organs [2]. Key energy-consuming organs include the brain, muscles, liver, kidneys, gastrointestinal tract, heart, and lungs [3,4].
Mitochondrial Function in the Cell
Mitochondria are not only involved in energy production but also have a plethora of functions including those mentioned below [5-7]:
- Calcium Homeostasis: Mitochondria play a vital role in regulating calcium homeostasis, and any perturbation of calcium levels leads to multiple diseases [8-10]. A more detailed look into calcium homeostasis and mitochondrial dysfunction can be found here.
- Apoptosis: It has been well established that mitochondria have a central role in regulating apoptosis [1].
- Fatty Acid Metabolism: Fatty acid oxidation takes place in the mitochondria [11]. Fatty acid metabolism provides energy for cells during high energy demand or when glucose levels are low [11].
- Heme Metabolism: Heme is a common and crucial metalloorganic cofactor necessary for almost all aerobic life [12]. Hemeproteins are the essential oxygen transporters with heme as a prosthetic group. Some of the critical hemeproteins include hemoglobin, myoglobin, neuroglobin, and hemocyanin. The mitochondria are the organelles where heme production is initiated and completed [12]. Heme is then transferred to apo-hemoproteins found throughout the cell or modified covalently.
- Reactive Oxygen Species (ROS) Regulation: One of the main by-products of aerobic respiration is ROS [13]. ROS are mainly produced in the mitochondria of cells. Mitochondria play an important role in maintaining cellular homeostasis by regulating ROS levels [14]. ROS can activate multiple signal transductions and play a vital role in aging. To learn more about the mitochondrial ROS theory of aging, read here.
- Cell Danger Response: The Cell Danger Response (CDR) is characterized as an evolutionarily conserved metabolic reaction triggered when a cell encounters severe threats that have the potential to harm or even lead to the cell's demise [15]. Mitochondria are the critical players in regulating and controlling CDR [16,17]. To learn more about the role of mitochondria in CDR, read here.
Mitochondrial Dysfunction in Diseases
These functional roles of the mitochondrion show that it is not only the powerhouse of the cell but the most important organelle of the cell. Hence, mitochondrial dysfunction causes many diseases, some of which are listed below:
- Chronic Fatigue: Fatigue is often characterized by a lack of energy, mental or physical tiredness, decreased endurance, and the need for prolonged recovery after physical activity [18]. Rest does not seem to alleviate this persistent and debilitating condition, which remains poorly understood despite its widespread prevalence [19]. Studies have found an association between fatigue and mitochondrial dysfunction, but more in-depth studies are required to get a clear understanding [18-21].
- Mitochondrial encephalomyopathy, lactic acidosis, and stroke-like episodes (MELAS) Syndrome: The energy needs of numerous organs with high energy demand, like the nervous system, skeletal and cardiac muscles, kidneys, liver, and endocrine system, are incapable of generating sufficient ATP to meet their needs when mitochondrial function is altered. One of the causes of mitochondrial dysfunction is mutation in mitochondrial DNA, leading to mitochondrial disorder [2,14,22,23]. MELAS syndrome is the name for common diseases caused by mitochondrial disorders [24]. Stroke-like episodes, dementia, epilepsy, lactic acidemia, myopathy, recurrent headaches, hearing impairment, diabetes, and short stature are common symptoms of multi-organ disease MELAS syndrome [24].
- Mitochondrial Cardiomyopathy: Key factors in the development of most heart failures are mitochondrial dysfunction and oxidative stress [5,25]. Mitochondrial cardiomyopathy refers to a heart-related condition marked by irregular heart muscle structure, function, or both. This arises due to genetic defects in the mitochondrial respiratory chain, without concurrent issues like coronary artery disease, hypertension, valvular disease, or congenital heart conditions [5,25-27].
- Neurodegenerative Disorders: Multiple neurodegenerative disorders like Alzheimer’s, Parkinson's, Huntington's, and autism are associated with mitochondrial dysfunction [28-34].
- Asthma: Chronic asthma is linked to mitochondrial dysfunction [35,36]. When allergens are present, the absorption of Ca2+ through the mitochondrial calcium uniporter (MCU) triggers an overabundance of ROS generation [36,37]. This excessive ROS production leads to the demise of lung epithelial cells, contributing to the breakdown of the epithelial barrier's function [35,38]. Changes in the entry of mitochondrial Ca2+ and heightened levels of cytosolic Ca2+ could amplify airway hyperactivity, a hallmark of asthma. As a result, both the MCU and other transporters that can disrupt mitochondrial Ca2+ balance present promising avenues for potential asthma treatments [35,37].
10 Natural Ways to Vitalize Mitochondrial Health
It is vital to keep your mitochondria hale and hearty to have a healthy lifestyle. There are many natural ways to boost mitochondrial function, ten of which are listed below:
- Yoga enhances the integrity of the mitochondria by modifying the expression of transcripts responsible for maintaining mitochondrial integrity [39]. This adjustment contributes to maintaining balanced free radical levels, a pivotal factor for elevating mitochondrial copy numbers. Moreover, it enhances cytochrome c oxidase (COX-II) activity, boosts nicotinamide adenine dinucleotide (NAD+) levels, optimizes markers of oxidative stress, and raises the levels of transcripts vital for preserving mitochondrial integrity [39,40]. Consequently, it diminishes the impact of disease activity on physical and mental well-being. As a result, yoga holds promise as a supplementary therapeutic approach in mitochondrial dysfunction [39-41].
- Calorie Restriction (CR) has been shown to increase mitochondrial biogenesis by 30% in a landmark study on male mice. The study found that implementing CR for durations of either 3 or 12 months triggered the expression of endothelial nitric oxide synthase and the formation of 3',5'-cyclic guanosine monophosphate in different tissues of male mice. This was accompanied by the generation of new mitochondria, leading to escalated oxygen consumption and ATP production. The study strongly provided proof that CR is responsible for increasing mitochondrial function [42]. Other studies have also found similar results in cell lines and animal models that CR induced the proliferation of the mitochondria via a signaling pathway involving peroxisome proliferation-activated receptor coactivator 1α [43].
- Intermittent Fasting’s (IF) role in improving mitochondrial function is known. A study found enhanced oxygen flow and ATP production through better mitochondrial respiration, demonstrated by strong respiratory control ratios in rats following IF for eight weeks with alternate day fasting (ADF) [44,45]. Another study observed reduced heart inflammation and oxidative stress, similar to younger rats following a 24-month ADF plan [44,45]. Combining ADF IF with intense intermittent exercise also improved metabolic pathways, significantly lowering malondialdehyde levels and plasma protein oxidation [45].
- Methylene Blue’s role in regulating mitochondrial function is well-documented [7]. Research has shown that methylene blue’s unique ability to redirect electrons within the mitochondrial electron transfer chain directly, from NADH to cytochrome c, results in increased complex IV activity, thereby boosting mitochondrial function and reducing oxidative stress [46,47]. These remarkable properties aid in curbing cell damage and enhancing cellular metabolism through mitochondrial improvements. Consequently, the impressive mitochondrial response of methylene blue has paved the way for exploring its potential applications in addressing conditions linked to mitochondrial dysfunction, including age-related issues affecting various tissues like the brain and skin [46-48]. To learn more about methylene blue, read here.
- Nicotinamide Riboside (NR) is a precursor of NAD+, and its supplementation is known to improve mitochondrial function. In a recent twin study, twenty pairs of monozygotic twins with differing body mass indexes were given increasing amounts of NR over five months. The authors found that supplementation led to enhancements in overall NAD+ metabolism, increased muscle mitochondria count, better myoblast differentiation, and improved gut microbiota balance in both twins of each pair [49]. Other studies have also found that NAD+ and NR play a vital role in improving mitochondrial function. To learn more about the functioning of niacin, read here.
- Curcumin is the most commonly found in turmeric and has antioxidant activities [50]. Studies have shown that curcumin can boost mitochondrial fusion activity, decrease mitochondrial fission mechanisms, and elevate mitochondrial biogenesis. Treatment with curcumin has demonstrated enhanced mitochondrial function and cell viability [50]. Curcumin potentially enhances mitochondrial potential and ATP levels and might also contribute to restoring mitochondrial fusion, likely through the upregulation of PGC-1α protein expression [50].
- Resveratrol is a polyphenolic phytoalexin in grapes, berries, peanuts, and wines [51]. It is recognized for its antioxidant, anti-inflammatory, anti-apoptotic, and anticancer properties [52]. Studies have found that resveratrol regulates mitochondrial function, redox processes, and dynamics. Additionally, resveratrol reduces mitochondrial damage caused by specific stressors [53]. For instance, it increases the presence of antioxidant enzymes within mitochondria, consequently reducing reactive species production by these structures [52,54]. Furthermore, resveratrol initiates mitochondrial growth, leading to improvements in the bioenergetic status associated with mitochondria in mammalian cells [51,52,54].
- Exercise plays a vital role in regulating mitochondrial functions. Studies have found that 30 minutes of daily exercise reduces mitophagy and increases longevity. A systematic review found that regular aerobic exercise enhanced mitochondrial morphology, biogenesis, dynamics, oxidative, and antioxidant capacity [55]. Thus, exercise remains our best behavioral medicine for improving mitochondrial health and individual longevity [56].
- Melatonin is a potent antioxidant that counters free radicals and ROS generated during cellular metabolism [25]. It protects mitochondria by neutralizing ROS, hindering the opening of the mitochondrial permeability transition pore, and activating uncoupling proteins [57]. Consequently, melatonin sustains the optimal membrane potential of mitochondria, ensuring their functions remain intact. Furthermore, melatonin governs the creation and movement of mitochondria. It frequently curtails the division of mitochondria while fostering their fusion. The rhythmic oscillation in mitochondrial dynamics mirrors melatonin's natural secretion cycle, observed particularly in pineal cells and likely in other cell types [58]. Recent studies also highlight melatonin's role in promoting mitophagy and enhancing mitochondrial equilibrium [58]. To learn more about melatonin, read here.
- Vitamin C is an essential nutrient for humans due to their inability to synthesize it from glucose [59]. It holds the capacity to enhance mitochondrial functioning. Its supplementation can mitigate excessive ROS production, safeguarding mitochondria and reducing inflammation across tissues, including skeletal muscle [59,60]. Consequently, it shows promise in enhancing recovery and athletic performance. However, the impact of antioxidant supplementation on skeletal muscle adaptation to exercise remains uncertain. While it might interfere with hormesis, where stress-induced ROS signal beneficial adaptations, it could also potentially prevent prolonged elevation of ROS caused by excessive exercise stress, leading to impaired function and damage [58-60].
+ Bonus!
Just Blue is 16 mg of pure pharmaceutical grade methylene blue, a compound known as an electron cycler, donating electrons to the electron transport chain and scavenging mitochondria and cytosol for free radicals and ROS. The result? Enhanced ATP production and enhanced antioxidant protection.
Conclusion
To have a long and healthy life, protecting the functionality of the mitochondria is of utmost importance. Mitochondria are not only the powerhouses of the cell but the most important organelles in your body. Longevity studies have shown that dysfunctional mitochondria are the root cause of numerous diseases, including neurodegenerative disorders, heart failure, and chronic fatigue. One can unleash cellular vitality by implementing natural ways to improve their mitochondrial health.
References
-
Bock, F. J. & Tait, S. W. G. Mitochondria as multifaceted regulators of cell death. Nat Rev Mol Cell Biol 21, 85–100 (2020).
-
Pizzorno, J. Mitochondria—Fundamental to Life and Health THE PATH AHEAD. Integrative Medicine: A Clinician’s Journal 13, 8–15 (2014).
-
Santulli, G., Xie, W., Reiken, S. R. & Marks, A. R. Mitochondrial calcium overload is a key determinant in heart failure. Proceedings of the National Academy of Sciences 112, 11389–11394 (2015).
-
Weissman, D. & Maack, C. Mitochondrial function in macrophages controls cardiac repair after myocardial infarction. J Clin Invest 133, (2023).
-
Chang, X. et al. Molecular mechanisms of mitochondrial quality control in ischemic cardiomyopathy. Int J Biol Sci 19, 426 (2023).
-
Yang, L., Youngblood, H., Wu, C. & Zhang, Q. Mitochondria as a target for neuroprotection: role of methylene blue and photobiomodulation. Transl Neurodegener 9, 1–22 (2020).
-
Tucker, D., Lu, Y. & Zhang, Q. From mitochondrial function to neuroprotection—an emerging role for methylene blue. Mol Neurobiol 55, 5137–5153 (2018).
-
Matuz-Mares, D., González-Andrade, M., Araiza-Villanueva, M. G., Vilchis-Landeros, M. M. & Vázquez-Meza, H. Mitochondrial calcium: effects of its imbalance in disease. Antioxidants 11, 801 (2022).
-
Chan, C. S., Gertler, T. S. & Surmeier, D. J. Calcium homeostasis, selective vulnerability and Parkinson’s disease. Trends Neurosci 32, 249–256 (2009).
-
Giorgi, C. et al. Mitochondrial calcium homeostasis as potential target for mitochondrial medicine. Mitochondrion 12, 77–85 (2012).
-
Jang, H.-S., Noh, M. R., Kim, J. & Padanilam, B. J. Defective mitochondrial fatty acid oxidation and lipotoxicity in kidney diseases. Front Med (Lausanne) 7, 65 (2020).
-
Swenson, S. A. et al. From synthesis to utilization: The ins and outs of mitochondrial heme. Cells 9, 579 (2020).
-
Silva, J. M., Wong, A., Carelli, V. & Cortopassi, G. A. Inhibition of mitochondrial function induces an integrated stress response in oligodendroglia. Neurobiol Dis 34, 357–365 (2009).
-
News, S. Mitochondria are the “canary in the coal mine” for cellular stress. Salk Institute https://www.salk.edu/news-release/mitochondria-are-the-canary-in-the-coal-mine-for-cellular-stress/ (2019).
-
Naviaux, R. K. Perspective: Cell danger response Biology—The new science that connects environmental health with mitochondria and the rising tide of chronic illness. Mitochondrion 51, 40–45 (2020).
-
Naviaux, R. K. Metabolic features of the cell danger response. Mitochondrion 16, 7–17 (2014).
-
Pradeu, T. & Cooper, E. L. The danger theory: 20 years later. Front Immunol 3, 1–9 (2012).
-
Naviaux, R. K. et al. Metabolic features of chronic fatigue syndrome. Proc Natl Acad Sci U S A 113, E5472–E5480 (2016).
-
Filler, K. et al. Association of mitochondrial dysfunction and fatigue: a review of the literature. BBA Clin 1, 12–23 (2014).
-
Naviaux, R. K. The Metabolic Features of Myalgic Encephalitis / Chronic Fatigue Syndrome ( ME / CFS ). 1–22 (2017).
-
Close, S. et al. The Economic Impacts of Myalgic Encephalomyelitis/Chronic Fatigue Syndrome in an Australian Cohort. Front Public Health 8, 1–8 (2020).
-
Wu, Z. et al. Mitochondrial DNA stress signalling protects the nuclear genome. Nat Metab 1, 1209–1218 (2019).
-
Ma, C., Xia, F. & Kelley, S. O. Mitochondrial Targeting of Probes and Therapeutics to the Powerhouse of the Cell. Bioconjug Chem 31, 2650–2667 (2020).
-
El-Hattab, A. W., Adesina, A. M., Jones, J. & Scaglia, F. MELAS syndrome: Clinical manifestations, pathogenesis, and treatment options. Mol Genet Metab 116, 4–12 (2015).
-
Wang, J., Toan, S., Li, R. & Zhou, H. Melatonin fine-tunes intracellular calcium signals and eliminates myocardial damage through the IP3R/MCU pathways in cardiorenal syndrome type 3. Biochem Pharmacol 174, 113832 (2020).
-
Gonzaga, C., Bertolami, A., Bertolami, M., Amodeo, C. & Calhoun, D. Obstructive sleep apnea, hypertension and cardiovascular diseases. J Hum Hypertens 29, 705–712 (2015).
-
Sequeira, V., Waddingham, M. T., Tsuchimochi, H., Maack, C. & Pearson, J. T. Mechano-energetic uncoupling in hypertrophic cardiomyopathy: Pathophysiological mechanisms and therapeutic opportunities. Journal of Molecular and Cellular Cardiology Plus 100036 (2023).
-
Lin, M. T. & Beal, M. F. Mitochondrial dysfunction and oxidative stress in neurodegenerative diseases. Nature 443, 787–795 (2006).
-
Bezprozvanny, I. & Mattson, M. P. Neuronal calcium mishandling and the pathogenesis of Alzheimer’s disease. Trends Neurosci 31, 454–463 (2008).
-
Abramov, A. Y., Potapova, E. V, Dremin, V. V & Dunaev, A. V. Interaction of oxidative stress and misfolded proteins in the mechanism of neurodegeneration. Life 10, 101 (2020).
-
Hamilton, J., Pellman, J. J., Brustovetsky, T., Harris, R. A. & Brustovetsky, N. Oxidative metabolism and Ca2+ handling in isolated brain mitochondria and striatal neurons from R6/2 mice, a model of Huntington’s disease. Hum Mol Genet 25, 2762–2775 (2016).
-
Anandatheerthavarada, H. K. & Devi, L. Amyloid precursor protein and mitochondrial dysfunction in Alzheimer’s disease. The Neuroscientist 13, 626–638 (2007).
-
Chan, C. S., Gertler, T. S. & Surmeier, D. J. Calcium homeostasis, selective vulnerability and Parkinson’s disease. Trends Neurosci 32, 249–256 (2009).
-
Panov, A. V et al. Early mitochondrial calcium defects in Huntington’s disease are a direct effect of polyglutamines. Nat Neurosci 5, 731–736 (2002).
-
Bhatraju, N. K. & Agrawal, A. Mitochondrial dysfunction linking obesity and asthma. Handbook of Mitochondrial Dysfunction 151–162 (2019).
-
Michaeloudes, C. et al. Molecular mechanisms of oxidative stress in asthma. Mol Aspects Med 85, 101026 (2022).
-
Mabalirajan, U. et al. Mitochondrial structural changes and dysfunction are associated with experimental allergic asthma. The Journal of Immunology 181, 3540–3548 (2008).
-
Reddy, P. H. Mitochondrial dysfunction and oxidative stress in asthma: implications for mitochondria-targeted antioxidant therapeutics. Pharmaceuticals 4, 429–456 (2011).
-
Gautam, S., Saxena, R., Dada, T. & Dada, R. Yoga—impact on mitochondrial health: clinical consequences. Ann Neurosci 28, 114–116 (2021).
-
Gautam, S., Kumar, U., Kumar, M., Rana, D. & Dada, R. Yoga improves mitochondrial health and reduces severity of autoimmune inflammatory arthritis: A randomized controlled trial. Mitochondrion 58, 147–159 (2021).
-
Gupta, S., Gautam, S., Kumar, U., Arora, T. & Dada, R. Potential role of yoga intervention in the management of chronic non-malignant pain. Evidence-Based Complementary and Alternative Medicine 2022, (2022).
-
Nisoli, E. et al. Calorie restriction promotes mitochondrial biogenesis by inducing the expression of eNOS. Science (1979) 310, 314–317 (2005).
-
López-Lluch, G. et al. Calorie restriction induces mitochondrial biogenesis and bioenergetic efficiency. Proceedings of the National Academy of Sciences 103, 1768–1773 (2006).
-
Real-Hohn, A. et al. The synergism of high-intensity intermittent exercise and every-other-day intermittent fasting regimen on energy metabolism adaptations includes hexokinase activity and mitochondrial efficiency. PLoS One 13, e0202784 (2018).
-
Savencu, C. E. et al. Impact of dietary restriction regimens on mitochondria, heart, and endothelial function: A brief overview. Front Physiol 12, 768383 (2021).
-
Atamna, H. et al. Methylene blue delays cellular senescence and enhances key mitochondrial biochemical pathways. The FASEB Journal 22, 703–712 (2008).
-
Xue, H., Thaivalappil, A. & Cao, K. The potentials of methylene blue as an anti-aging drug. Cells 10, 3379 (2021).
-
Poteet, E. et al. Neuroprotective actions of methylene blue and its derivatives. PLoS One 7, e48279 (2012).
-
Lapatto, H. A. K. et al. Nicotinamide riboside improves muscle mitochondrial biogenesis, satellite cell differentiation, and gut microbiota in a twin study. Sci Adv 9, eadd5163 (2023).
-
Tabassum, H. & Parvez, S. Curcumin and mitochondria. in Mitochondrial Physiology and Vegetal Molecules 439–454 (Elsevier, 2021).
-
Shakibaei, M., Harikumar, K. B. & Aggarwal, B. B. Resveratrol addiction: to die or not to die. Mol Nutr Food Res 53, 115–128 (2009).
-
Jardim, F. R. et al. Resveratrol and brain mitochondria: a review. Mol Neurobiol 55, 2085–2101 (2018).
-
Ahmed, T. et al. Resveratrol and Alzheimer’s disease: mechanistic insights. Mol Neurobiol 54, 2622–2635 (2017).
-
Albani, D., Polito, L., Signorini, A. & Forloni, G. Neuroprotective properties of resveratrol in different neurodegenerative disorders. Biofactors 36, 370–376 (2010).
-
Lim, A. Y., Chen, Y.-C., Hsu, C.-C., Fu, T.-C. & Wang, J.-S. The effects of exercise training on mitochondrial function in cardiovascular diseases: A systematic review and meta-analysis. Int J Mol Sci 23, 12559 (2022).
-
Memme, J. M., Erlich, A. T., Phukan, G. & Hood, D. A. Exercise and mitochondrial health. J Physiol 599, 803–817 (2021).
-
Saldmann, F., Viltard, M., Leroy, C. & Friedlander, G. The naked mole rat: A unique example of positive oxidative stress. Oxid Med Cell Longev 2019, (2019).
-
Tan, D.-X., Manchester, L. C., Qin, L. & Reiter, R. J. Melatonin: a mitochondrial targeting molecule involving mitochondrial protection and dynamics. Int J Mol Sci 17, 2124 (2016).
-
Kc, S., Càrcamo, J. M. & Golde, D. W. Vitamin C enters mitochondria via facilitative glucose transporter 1 (Gluti) and confers mitochondrial protection against oxidative injury. the FASEB Journal 19, 1657–1667 (2005).
-
Gonzalez, M. J., Miranda-Massari, J. R. & Olalde, J. Vitamin C and mitochondrial function in health and exercise. in Molecular Nutrition and Mitochondria 225–242 (Elsevier, 2023).
Comments (0)
There are no comments for this article. Be the first one to leave a message!